Researchers have made progress in precision timekeeping by using thorium-rich crystals and a type of laser called a frequency comb in a vacuum chamber.Credit: Ye Labs, JILA, NIST and Univ. Colorado
What do you get when you put a nuclear physicist, an optical physicist and a crystal-growing maestro together in a laboratory? This is not the beginning of a bad joke, but the starting point for making what could become the world’s most accurate timepiece. The level of boundary-crossing innovation required to make a nuclear clock1 serves as an example of how cooperation can exist alongside competition in science.
Atomic clocks currently hold the world record for most accurate timekeeping. They tick out time through the frequency of light emitted and absorbed by electrons jumping between atomic energy levels. Lasers that are locked to a clock’s frequency provide the read-out.
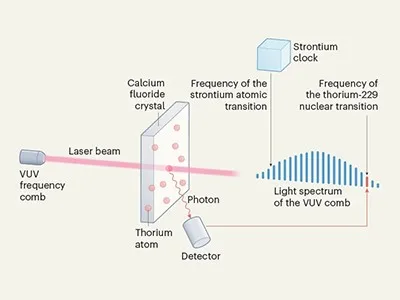
Countdown to a nuclear clock
Nuclear clocks, by contrast, tap into the energy shifts of protons and neutrons inside an atom’s nucleus. These energy transitions occur at a higher frequency than do those of electrons, making for a faster tick that could someday slice time more finely than atomic clocks can. And nuclear clocks come with other benefits. They are inherently more robust than atomic ones, because the nucleus and its energy transitions are more impervious to external perturbations than orbiting atomic electrons are. The transitions are also more heavily influenced by constants of nature, such as the strength of the strong nuclear force. This makes nuclear clocks highly sensitive instruments for testing fundamental physics. But nuclear clocks are also difficult to make, which is why it has taken almost 50 years to get close to a working model. For most atoms, a huge amount of energy is needed to lift the nucleus away from its lowest energy state, well beyond what can be achieved using precise, stable probing lasers.
In 1976, patterns in the radioactive decay of the isotope thorium-229 suggested that the energy gap between the nucleus’s ground state and its first excited state is much smaller than for other atomic nuclei2. Further calculations indicated that the window of energy in which the transition occurs might be accessible to lasers, leading physicists in 2003 to propose a thorium-based clock3.
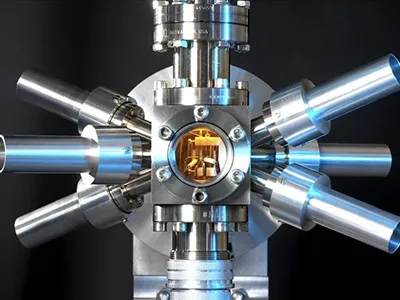
Best ever clocks: breakthrough paves way for ultra-precise ‘nuclear’ timekeepers
But theoretical models of the nucleus cannot predict the energy of such transitions with the necessary accuracy, so finding this one meant searching an enormous range of possible values. Moreover, thorium-229 decays slowly from its first energy state. So, with a half-life of around 30 minutes, the chance of observing the decay from second to second is low.
This wearisome work has received a new impetus in the past few years — thanks in no small part to input from scientists in fields beyond atomic and nuclear physics, as Adriana Pálffy and José Crespo López-Urrutia write in a News and Views article. Last year, rare-isotope specialists at CERN, Europe’s particle-physics laboratory near Geneva, Switzerland, observed the low-energy transition for the first time by making thorium-229 using an innovative method4,5. At the same time, solid-state physicists at the Technical University of Vienna grew crystals embedded with so many thorium-229 atoms that the decay became visible amid other nuclear processes4.
Researchers realized that the experimental problems were too diverse for any one group to solve alone, says Ekkehard Peik, a physicist at the PTB, Germany’s national metrology institute in Braunschweig. Peik’s team6 was one of two6,7 to stimulate the energy transition earlier this year. “The community — different groups — had to solve the different problems, one by one,” he says. The international Thorium Nuclear Clock project, which is funded by the European Research Council, was explicitly designed to bring together the necessary expertise.
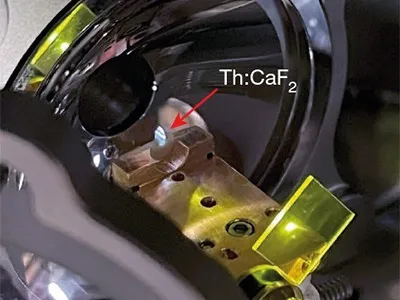
Read the paper: Frequency ratio of the 229mTh nuclear isomeric transition and the 87Sr atomic clock
Now, optical physicist Jun Ye at JILA, a research institute in Boulder, Colorado, and his colleagues have built on these advances1. They not only succeed in stimulating the transition in thorium-229 with exquisite precision, but also hook the thorium up to another timekeeper using a type of laser known as a frequency comb. This device, which creates a spectrum of equally spaced frequency lines at higher energies than ever before, has been used for measurements since 20128. Ye and his co-authors saw its potential for driving a nuclear clock but were able to do so only when other groups had found ways to boost the thorium-229 signal in crystals and shown where in the spectrum to look.
As such, this nuclear clock is a great example of the merits of reaching beyond silos. But, like many areas of science, the field of precision clocks thrives on competition as well as cooperation. There are many other avenues to explore, such as whether studying a small number of thorium-229 ions in a trap will provide a more stable environment in which to observe the transition than the crystal does. Laser physicists, too, might scramble to find other ways to improve the high-frequency devices needed for this extreme transition. Despite all this, a practical working nuclear clock has yet to be made. But that will now be only a matter of time.