Abstract
Unravelling abrupt alterations in the gut microbiota of wild species associated with nutritional stress is imperative but challenging for wildlife conservation. This study assessed the nutritional status of wild red deer during winter on the basis of changes in faecal nitrogen (FN) and urea nitrogen/creatinine (UN: C) levels and identified gut microbes associated with nutritional status via nutritional control experiments and metagenomic sequencing. The FN of wild red deer in winter 2022 was significantly lower than that in winter 2021 (p < 0.05, winter 2021: 1.37 ± 0.16% and winter 2022: 1.26 ± 0.22%), and the UN: C ratio increased (winter 2021: 2.19 ± 1.65 and winter 2022: 3.05 ± 3.50). Similar trends were found in late winter, which indicated greater nutritional pressure in winter (2022) and late winter. Compared with winter 2021, abundances of Ructibacterium and Butyrivibrio significantly increased, and Acetatifactor and Cuneatibacter significantly decreased during winter 2022 (p < 0.05). Compared with early winter, the cell growth and death pathways increased and lipid metabolism and its subpathway of secondary bile acid synthesis (ko00121) significantly decreased during late winter (p < 0.05), which was similar to the changes in malnourished experimental red deer. Abrupt alterations in the gut microbiota should receive increased attention when monitoring the nutritional health of wild ungulates. This study provides new insights and critical implications for the conservation of wild ungulate populations.
Introduction
Changes in nutritional status embody the adaptation of wild ungulates to their habitat and are the key to maintaining the health and stability of the population1. In the northern forests of China, wild ungulates may suffer from varying degrees of nutrient deficiency due to low temperatures and low plant resource quality in winter2,3,4 and their health and survival are greatly threatened. Assessing nutritional status is helpful in understanding the adaptability of wild ungulates and is an important scientific basis for formulating conservation measures for wild ungulate populations.
As a non-invasive sampling method, the assessment of nutritional status using urine metabolites shows high performance and meets the requirements of wildlife conservation and is a common method for assessing the nutritional status of wild ungulate populations in winter2,3,4,5. A urine urea nitrogen/creatinine (UN: C) ratio exceeding 4 (UN: C > 4) appropriately indicates the nutrient deficiency of ungulates, and accelerated endogenous protein breakdown leads to an increased concentration of urea nitrogen in urine6.
Gut microbes play an important role in maintaining nutritional balance and can provide important nutritional information7. In human and mouse models, nutritional status issues can lead to abrupt changes in gut microbes8,9. Gut microbes may mutate abruptly or persistently change in the early stages of nutritional deficiency10. Significantly decreased microbial diversity and abrupt changes in microbial functions were observed in nutritionally deficient sheep11, which suggests links between nutritional status and the gut microbes of ungulates. The increasing recognition of microbiome responsiveness highlights the hypothesis that specific microbial communities can change with the nutritional status of organisms. Therefore, gut microbes may be indicative of the nutritional stress that wild ungulates encounter, and there may be certain correlations with changes in the UN: C of wildlife.
Red deer (Cervus elaphus), which is a Class II key protected wildlife in China, serves as the main prey for rare carnivores, such as the Amur tiger (Panthera tigris altaica), and maintains the stability of the forest ecosystem12,13. However, wild red deer populations suffer from greater nutritional stress in winter because of the harsh environment2,14. Cervids mainly focus on alterations in gut microbes affected by season-, geographical location-, and captivity- related dietary changes15,16,17,18, whereas red deer mainly focus on the associations between the environment and gut microbes and the microbial differences between species19,20. Although studies highlight the urgency and importance of identifying key microbial members that maintain and respond to the health of ungulate hosts21, few studies have examined the relationship between gut microbes and the nutritional health of red deer. In this study, we compared changes in the nutritional status of wild red deer between different years and periods of winter on the basis of faecal nitrogen (FN)22 and urea nitrogen/creatinine (UN: C)6 levels to explore potential microbial populations and functions related to nutritional status in wild red deer via nutritional experiments and metagenomic sequencing. This study provides critical information for understanding the health of wild red deer populations in the winter, and has important theoretical implications for protecting and managing wild ungulate populations.
Materials and methods
Study area
The Gaogesitai National Nature Reserve is located in the southern Greater Khingan Mountains of China with a total area of 1062.84 km2. The vegetation in the protected area is largely mountain forest mainly consisting of Ulmus pumila, Quercus mongolica, Salix rosmarinifolia, and Ostryopsis davidiana. Owing to the special geographical characteristics of the reserve, the annual climate change is obvious. The average temperature in winter can drop below − 16.0 °C, the extreme minimum temperature can drop below − 42.0 °C, and the longest number of continuous snow days can reach 100. Harsh environmental conditions in winter exert greater survival pressure on wild red deer.
Collection of faeces and snow urine samples
In the winters of 2021 (12/2021–02/2022) and 2022 (12/2022–02/2023), fresh faecal samples and snow urine samples from wild red deer were collected in the Gaogesitai National Nature Reserve by tracking footprint chains. Faecal and snow urine samples were collected using disposable polyethylene (PE) gloves, and new PE gloves were replaced each time to prevent cross-contamination between samples. Snow-contaminated faecal particles were avoided to prevent snow accumulation from affecting microorganisms in the samples. When the snow urine samples were collected, only the middle part of the snow urine (yellow‒brown parts) was collected, and snow around the urine stain was avoided. After samples were collected, 4–5 drops of 10% dilute sulfuric acid were added to the snow urine samples to avoid degradation during transportation. For field collection, only one faecal sample and snow urine sample were collected from each footprint chain to minimize individual duplication. A total of 43 fresh faecal samples and 60 fresh urine samples were collected (winter 2021: 20 faecal samples and 30 urine samples; winter 2022: 23 faecal samples and 30 urine samples).
Nutritional control experiments
Two healthy male and female captive red deer of similar body size and age were selected as the experimental subjects (male numbers: Male-1 and Male-2; female numbers: Female-1 and Female-2). In accordance with previous studies23,24, Quercus mongolica, Ulmus pumila, Salix rosmarinifolia, Ostryopsis davidiana, Prunus sibirica and mixed herbs were selected for this experiment as the main food resources to ensure similar feeding with wild red deer. Collected plants were not manually processed and were fed freely to experimental red deer. During the preexperimental phase, the actual daily consumption of experimental individuals was determined on the basis of the daily food supply and surplus amount (Supplementary Table S1). This experimental portion lasted for 30 days, including 10 days in the preexperimental phase and 20 days in the formal experimental phase, and this experimental portion was defined as the low-protein low-energy feeding period (LP-LE).
At the end of the above experimental portion, the next portion of the experiment was started. In this experimental portion, the actual daily consumption of the four red deer was reduced by a quarter and plant species were kept unchanged (Supplementary Table S2). This experimental portion lasted for 10 days and was defined as the limited feeding period (LF).
The experimental periods of LP-LE and LF correspond to insufficient nutrient intake and food resource shortage in the wild, respectively. At the end of each experimental portion, 10 mL of fresh blood was collected from each red deer and centrifuged at 3000 RPM for 10 min. The serum was used to measure the concentrations of serum urea nitrogen (SUN), glucose (GLU), triiodothyronine (T3) and thyroxine (T4), and then, the nutritional status of the experimental red deer was evaluated.
Metagenomic sequencing
Total DNA was extracted via the Mag-Bind Soil DNA Kit (OMEGA, USA). The concentration and purity of the extracted DNA were tested via Qubit 4 fluorometer (Invitrogen, USA), and the quality of the extracted DNA was measured by 1% agarose gel electrophoresis. DNA concentrations exceeded the minimum sequencing requirement (> 2.5 ng/µL), and the total amount of DNA at least met the requirements for single sequencing (> 0.2 µg), which indicated that the DNA quality fully met the requirements for subsequent analysis. A library was constructed via the Illumina TruSeq Nano DNA LT Library Preparation Kit (Illumina, USA), and double-ended sequencing was performed via the Illumina NovaSeq™ X Plus sequencing platform (2 × 150 bp).
The original sequence was screened and filtered via fastp software (v0.23.2) to remove certain low-quality sequences. Then, sequences with lengths < 50 bp and those containing fuzzy bases were removed. Fastp software (v0.23.2) was used to calculate quality statistics for the sequencing data after quality control. The base mass of the sequences used in this study was greater than 20, which met the quality requirements for subsequent analysis (Supplementary Figure S1). Minimax2 software (v2.24-R1122) was used to compare the effective sequences with the host sequences (host sequence: https://www.ncbi.nlm.nih.gov/datasets/genome/GCF_910594005.1/), and sequences with a mapping score greater than 10 with the host genome were discarded. Valid reads from which the host contaminating sequence was removed were used for subsequent analysis.
Data processing and analysis
Repetitive sequences in the valid read sequences were removed via minimap2 software (v2.24-R1122), and the number of reads aligned with the gene sequence was counted via featureCounts software (v2.0.3), which considers the read count (RC) of each gene sequence. On the basis of the non-redundant gene sets and RCs, the GTDB database (release 207), NCBI-nt database (v2022-11-01) and RVDB database (v24.1) were integrated with Kraken2 software (v2.0.8-beta) to construct the Kraken2 database for annotation. Protein sequences were compared with the KEGG functional database (v2020-09-20) via the MMseqs2 software (v13.45111) search module to annotate microbial functions.
The Kruskal‒Wallis test was used to examine differences in FN and UN: C in different years and during different winter periods. Box plots and bar plots were generated with R software (v4.3.2) to show the distribution of UN: C and the microbial composition of wild red deer in winter25. PLS-DA was used to describe differences in the gut microbial structure and function. The Wilcoxon rank-sum test was used to identify microbial communities and functional pathways with different abundances in red deer between different years and periods of winter and between different experimental phases. On the basis of changes in the gut microbes of the experimental red deer at different phases, gut microbes with the same changes as those of the wild red deer were defined as potential microbial groups that respond to nutritional status, and bar plots were used to show the common change characteristics of gut microbes between experimental and wild red deer. The significance threshold for statistical analysis was 0.05, and the Benjamini‒Hochberg procedure was used for multiple corrections of the p value.
Results
Differences in FN and UN: C in wild red deer during winter
The FN of wild red deer in the winter of 2022 was 1.26 ± 0.22% while in the winter of 2021 it was 1.37 ± 0.16%. Compared with the winter of 2021, the FN of wild red deer in the winter of 2022 significantly decreased (p < 0.05, Fig. 1). FNs of wild red deer were 1.25 ± 0.16 in the early winter of 2022, 1.11 ± 0.27 in the late winter, 1.47 ± 0.10 in the early winter of 2021, and 1.27 ± 0.15 in the late winter. Compared with early winter, the FN of wild red deer in late winter was significantly lower (p < 0.05, Fig. 1).
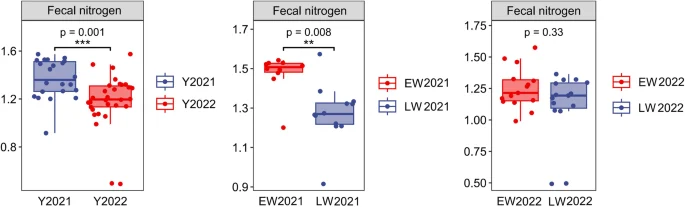
Differences in FNs of wild red deer in winter (Y = year, EW = early winter, LW = late winter).
The UN: C ratio was 3.05 ± 3.50 for wild red deer in the winter of 2022 and 2.19 ± 1.65 in the winter of 2021. The UN: C ratio was 3.31 ± 2.14 in late winter of 2022, 2.80 ± 4.55 in early winter, 2.49 ± 1.97 in late winter of 2021, and 1.90 ± 1.24 in early winter (Fig. 2a). Compared with winter 2021 and early winter, the UN: C ratio in wild red deer increased during winter 2022 and late winter (Fig. 2a). Combined with the nutritional threshold (UN: C > 4), the proportion of urea samples with UN: C > 4 in wild red deer during the winter of 2022 was 23.33% with 20.00% in late winter and 3.33% in early winter. The proportion of snow urea samples with UN: C > 4 in wild red deer during the winter of 2021 was 10.00%, 6.67% were in late winter, and 3.33% were in early winter (Fig. 2b).
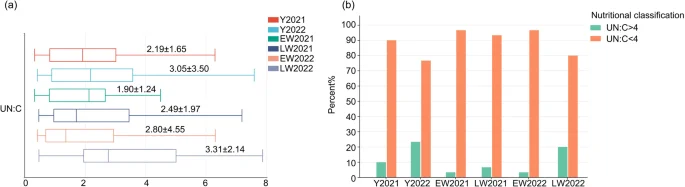
Changes in nutritional indicators of wild red deer in winter (a. the range of UN: C of wild red deer during winter; b. proportion of urine samples with UN: C exceeding the nutrient threshold; Y = year, EW = early winter, LW = late winter).
Compared with those in the winter of 2021, the FN of wild red deer in the winter of 2022 was low, the UN: C ratio was high, and the number of urine samples with a UN: C ratio > 4 accounted for a large proportion of the total sample, which indicated relatively low nitrogen intake and poor nutritional status of wild red deer in the winter of 2022.
Characteristics and differences in gut microbes of wild red deer
The composition of gut microbes of wild red deer in the winter were mainly Firmicutes and Bacteroidetes (Fig. 3a). At the genus level, 3 genera, namely Pseudoflavonifractor, Clostridium, and Bacteroides, were the most abundant taxa followed by Ruminococcus, Alistipes, and Oscillibacter (Fig. 3b). On the basis of results of the PLS-DA, at the genus level, the microbiota analysed on the basis of faecal samples clustered discretely in a PCo plot (PCo1 = 11%, PCo2 = 6.6%), which indicated that the microbial structure of wild red deer differed between the winter of 2021 and 2022 (Fig. 3c).
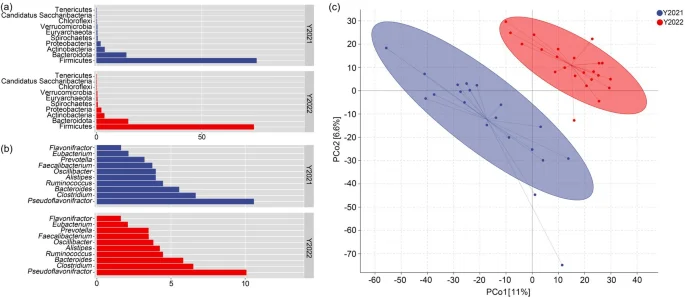
Composition and structure of gut microbes in wild red deer during winter (a. the microbial composition at the phylum level; b. the microbial composition at the genus level; c. the microbial structural differences at the genus level).
Compared with the winter of 2021, abundances of Christensenella, Parabacteroides, Ructibacterium, Phocaeicola, Butyrivibrio, Roseburia, and Prevotella significantly increased in the winter of 2022 (p < 0.05, Fig. 4a). Compared with early winter, the abundance of Sarcina in late winter significantly increased, and abundances of Clostridium, Acetatifactor and Cuneatibacter significantly decreased (p < 0.05, Fig. 4b).
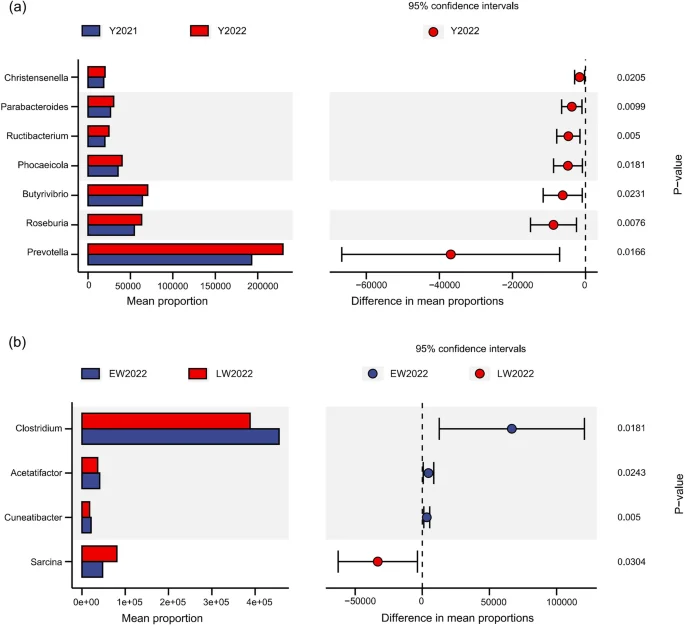
Differences in abundances of gut microbes in wild red deer (a. differential gut microbes during different years of winter; b. differential gut microbes during different periods of winter; Y = year; EW = early winter, LW = late winter).
At the microbial functional level, the microbial functions of wild red deer in winter were mainly metabolism and genetic information processing, which was followed by cellular processing, disease, environmental information processing and organismal systems (Fig. 5a). Functional subpathways were mainly composed of metabolic pathways including carbohydrate metabolism, amino acid metabolism, and metabolism of cofactors and vitamins (Fig. 5b). Results of PLS-DA revealed that the microbial functional structure of wild red deer was relatively discrete between the 2021 and 2022 winters (PCo1 = 51.8%, PCo2 = 8.2%) at the KEGG L2 level, which indicated that the microbial functional structure of wild red deer differed between 2021 and 2022 winters (Fig. 5c).
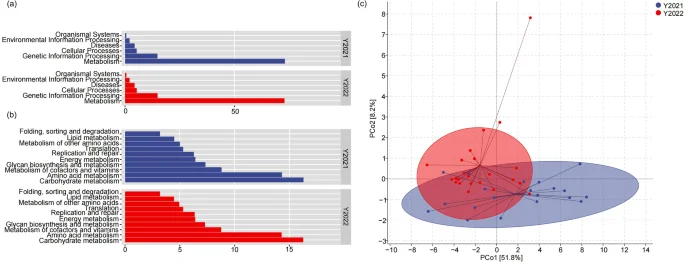
Functional composition and structure of gut microbes in wild red deer during winter (a. functional composition at the KEGG L1 level; b. functional composition at the KEGG L2 level; c. functional structure differences at the KEGG L2 level).
Compared with the winter of 2021, microbial functions involved in cellular processes significantly increased in the winter of 2022 (p < 0.05, Fig. 6a). Compared with that in early winter, microbial function related to cell growth and death significantly increased in late winter, whereas lipid metabolism significantly decreased (p < 0.05, Fig. 6b).
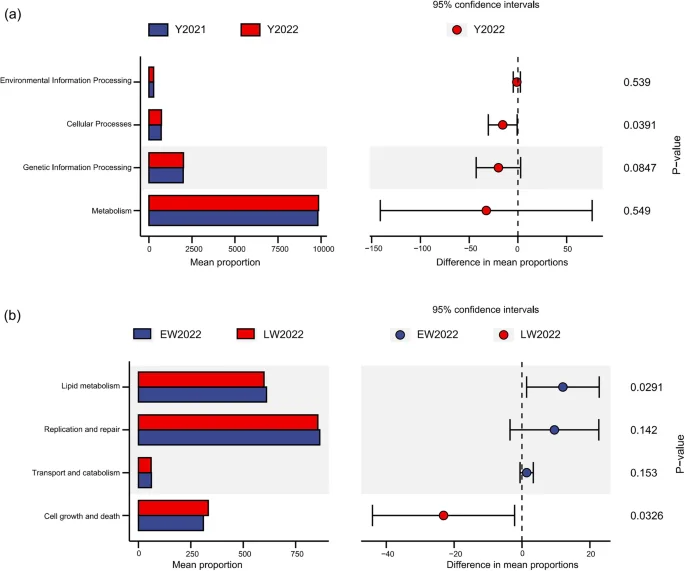
Differences in microbial functions of wild red deer during winter (a. between different years of winter; b. between different periods of winter; Y = year, EW = early winter, LW = late winter).
Changes in the serum physiological indices and nutritional status of experimental red deer
The nutritional status of the experimental red deer was evaluated to clarify the relationship between gut microbes and nutritional status. In the LP-LE phase, the SUN content increased, the GLU content tended to decrease, and the SUN content of Male-2 and Female-1 exceeded the nutritional threshold (SUN > 20 mg/dL), which indicated certain nutritional issues in the LP-LE phase.
In the LF phase, levels of SUN, GLU, T3 and T4 in the experimental red deer noticeably decreased, and the SUN content in all experimental individuals exceeded the nutritional threshold (Table 1), which indicated a persistent decline in nutritional status after further feeding restrictions. Overall, the experimental red deer were malnourished.
Association of gut microbes with the nutritional status of wild red deer
In the LP-LE phase, abundances of Christensenella, Ructibacterium and Butyrivibrio in the experimental red deer increased, and changes in the abundances of Ructibacterium and Butyrivibrio were completely consistent with those in wild red deer in the winter of 2022 (Figs. 4a and 7a). In the LF phase, the abundance of Butyrivibrio in the experimental red deer increased, which was consistent with the pattern observed for wild red deer in the winter of 2022 (Figs. 4a and 7b). Overall, the abundances of Acetatifactor and Cuneatibacter decreased, which was consistent with the pattern observed for the wild red deer during late winter (Figs. 4b and 7b).
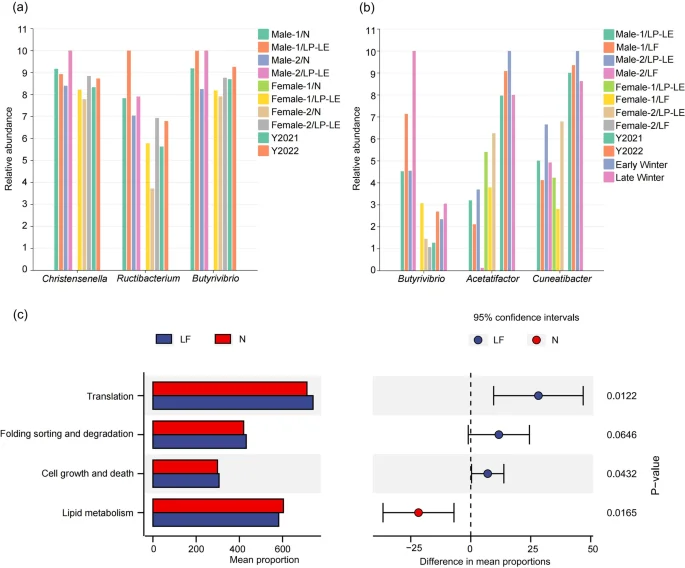
Commonality analysis of gut microbes between experimental and wild red deer (a. gut microbes with common changes between experimental red deer in the LP-LE phase and wild red deer; b. gut microbes with common changes between experimental red deer in the LF phase and wild red deer; c. functional pathways with differences in expression between different experimental phases; N = normal feeding, LP-LE = low-protein and low-energy feeding period, LF = limited feeding period).
At the microbial functional level, the cell growth and death pathway significantly increased in the experimental red deer, whereas the lipid metabolism pathway significantly decreased (p < 0.05, Fig. 7c), which was consistent with the microbial functional changes in wild red deer during late winter (Fig. 6b). Further research revealed that ko00121 exhibited the same changes in late winter and during the limited feeding phase with a significant decrease in expression (p < 0.05, Fig. 8).
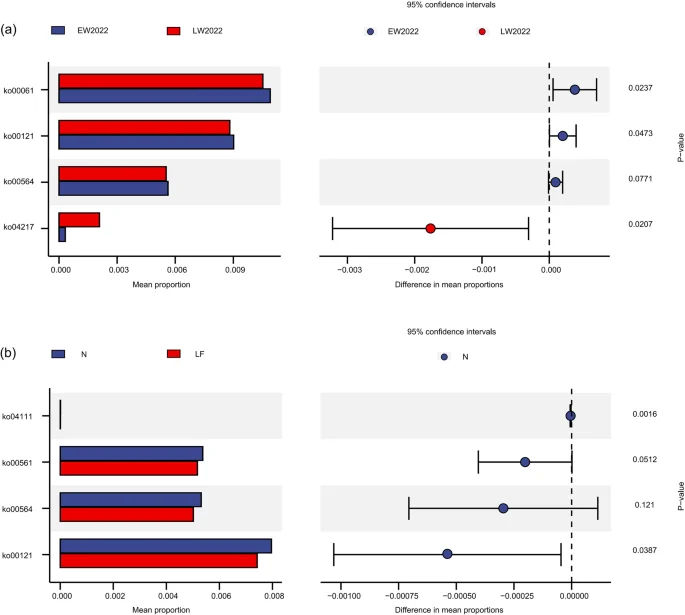
Differential analysis of the gut microbial functional subpathways in red deer (a. in wild red deer during different periods of winter; b. in experimental red deer during different phases of nutritional control experiments; EW = early winter, LW = late winter, N = normal feeding, LF = limited feeding).
Discussion
Changes in the FN and UN: C values of wild red deer in winter
The crude protein content of available plants is the most critical limiting nutrient for wild red deer in winter26. Studies have shown that faecal nitrogen (FN) is related to the intake of crude protein, which can reflect the quality of crude protein in food resources22. In this study, all faecal samples were collected from the same region and patches, and the FN value could objectively reflect the crude protein intake of wild red deer without regional bias4. These results revealed that the FN values of wild red deer in the winter of 2022 and late winter were significantly lower than those in the winter of 2021 and early winter, respectively, which indicated that the quality of available plants in the winter of 2022 and late winter was worse and caused the intake of crude protein in wild red deer to decline.
Inadequate crude protein intake may lead to endogenous protein degradation and increased UN: C values6. In this study, compared with those in the winter of 2021 and early winter, the UN: C values of wild red deer increased in the winter of 2022 and late winter, which indicated a metabolic relationship between inadequate crude protein intake and UN: C values. A UN: C value exceeding 4 appropriately implies nutrient deficiency6. Seven snow urine samples had UN: C > 4 in the winter of 2022 (23.33%), of which six samples were collected in late winter, and this indicates that the nutritional deficiency of wild red deer individuals in late winter might be more serious. Although nutritional assessment using UN: C values has certain limitations27,28, rising UN: C values in winter imply a deterioration in nutritional status3,4. The increased UN: C values in the winter of 2022 and late winter reflect the greater nutritional stress faced by wild red deer.
Characteristics of gut microbes in wild red deer during winter
Firmicutes and Bacteroidetes constitute the main microbial communities of wild red deer in winter, and are mainly related to feeding in the natural environment29. Microbial characteristics were consistent with those of previous studies on gut microbes of red deer30,31 and revealed a microbial structure similar to that of roe deer32, sika deer33, and moose34, which indicated that Firmicutes and Bacteroidetes were superior in cervids. Firmicutes can produce a series of decomposition enzymes to decompose and utilize plants, especially those that degrade cellulose35. Many macromolecular-degrading microflora of Bacteroides species can effectively decompose crude proteins and carbohydrates36. The high abundance of Firmicutes and Bacteroidetes helps wild red deer process plant resources in the natural environment and is an ecological adaptation of cervids to feeding conditions in the wild. In addition, a quantitative relationship between the abundance of Firmicutes and Bacteroidetes was observed, which resulted in higher Firmicutes/Bacteroidetes (F/B) ratio in winter. The quantitative relationship between gut microbes is related to the lower contents of crude protein, starch and lactate in limited foods that are available in winter16, and high F/B ratios are associated with higher fermentation efficiency and promote energy gain from these limited food resources37. Moreover, gut microbes with high F/B ratios can facilitate fat deposition in wild red deer38. Therefore, a microbial structure with high F/B ratios is essential for adapting to harsh environments in winter.
The main microbial genera in wild red deer are Firmicutes and Bacteroidetes. Clostridium is involved in the digestion of complex polysaccharides and structurally simple carbohydrates39. Ruminococcus, which is the main cellulose-degrading bacterium in Firmicutes, can degrade cellulose in plants to produce acetate, succinate, and ethanol40. Faecalibacterium, Eubacterium, and Prevotella are involved in the polysaccharide composition of foods and can encode primary fermentation enzymes for the digestion of dietary polysaccharides41,42,43. Bacteroides and Alistipes play important roles in carbohydrate and energy metabolism44,45 and exert critical influences on maintaining the body temperature of wild red deer. The core microflora in wild red deer is important for promoting nutrient metabolism and energy capture. Maintaining the relative stability of the core microflora is essential for the ecological adaptation of wild red deer.
However, some differences in microbial composition and function were found between the winters of 2021 and 2022. Owing to the similar structure of plant resources, microbial differences in wild red deer might be related to other factors. Physiological conditions are considered another important factor that contributes to changes in gut microbes46. In terms of FN contents and UN: C values, microbial differences between the winter of 2021 and 2022 may be related to differences in nutritional stress faced by wild red deer.
Response of gut microbes to the nutritional status of red deer
Considering limitations of UN: C in the nutritional assessment of ungulates, a series of studies are urgently needed to broaden indicators and systems of nutritional evaluation. Therefore, this study focused on the response characteristics of gut microbes to changes in nutritional stress in red deer. Nutritional stress in wild red deer in winter can be attributed to two factors as follow: poor quality and a shortage of available plants. To further identify relationships between changes in gut microbes and nutritional status, this study selected captive red deer to simulate the nutrient stress faced by red deer in the wild.
In the LP-LE phase, the GLU content in the experimental red deer decreased and the SUN content increased, which indicated that low-protein and low-energy feeding caused nutritional pressure on experimental red deer. The SUN content of Male-2/LP-LE and Female-1/LP-LE individuals exceeded 20 mg/dL, which implies that endogenous protein decomposition caused an increase in the concentration of SUN6. In the LF phase, the GLU content further decreased and the SUN concentration exceeded 20 mg/dL, which indicated that all experimental individuals were threatened by nutritional deficiency and endogenous protein decomposition accelerated6,47. Indices of T3 and T4 can reflect the metabolic level and physiological changes of ungulates in the short term48,49. In the LF phase, the decrease in contents of T3 and T4 also indicated that the nutritional status of experimental red deer deteriorated.
Combined with changes in gut microbes, in the LP-LE and LF phases, the abundance of some bacterial genera changed and was consistent with that of wild red deer. In related studies, decreases in Christensenella and Butyrivibrio abundances were associated with poor food quality and weight loss50,51. Ructibacterium is a new rod-shaped bacterium that has been poorly studied. However, the genus has been classified into the validly named family Oscillospiraceae52, and a significant increase in the abundance of Oscillospiraceae was found in malnourished sheep11. Therefore, we speculated that the deterioration of nutritional status was responsible for the increased abundance of Ructibacterium in the experimental and wild red deer. In addition, the isolation of gut microbes from mice receiving a high-calorie diet revealed high levels of Acetatifactor53. Therefore, low-energy feeding might cause a decrease in the abundance of Acetatifactor in experimental and wild red deer.
In terms of microbial functions, wild red deer presented the same changes as the experimental red deer and showed a significant increase in cell growth and death pathways and a significant decrease in lipid metabolism pathways. Persistent low temperatures and poor food availability might shorten the cell cycle and increase the risk of cytopathy, which thereby stimulates cell growth and death pathways. Further results revealed that the level of lipid metabolism function was mainly related to the secondary bile acid synthesis subpathway. Primary bile acids are synthesized from cholesterol in the liver54 and are stimulated by dietary intake and excreted into the intestinal lumen, where they are further synthesized into secondary bile acids by gut microbes (Figure S2)55,56. By forming micelle complexes, secondary bile acids can promote the dissolution, digestion, and absorption of lipids57. When faced with poor-quality food resources, the ability of red deer to obtain fat from food is limited and greater nutritional pressure leads to rapid depletion of body fat. A lack of fat sources may affect the liver‒intestine cycle in red deer and thereby reduce the level of secondary bile acid synthesis. Furthermore, the weakening of secondary bile acid synthesis from nutritional issues suggests that we should pay attention to abnormal liver function. Nutritional status problems may cause a decline in liver metabolic capacity, such as liver cell damage and reduced liver function58, and directly affects the synthesis of secondary bile acids. Therefore, further research should focus on the liver function and metabolite synthesis of nutritionally challenged red deer. The short duration of this nutritional control experiment may have limited our understanding of changes in gut microbes. Further research should include longitudinal time series experiments that track changes in gut microbes of red deer under different nutritional stresses.
Conclusion
This study revealed a dominant community consisting of Firmicutes and Bacteroidetes in wild red deer during winter, which emphasizes the importance of high F/B ratios in red deer adaptation to the wild environment. The environmental stress caused by a low quality of plant resources and limited food availability could cause changes in the nutritional status of wild red deer and the gut microbial response. More attention should be given to abrupt changes in the genera Ructibacterium, Butyrivibrio, Acetatifactor, and Cuneatibacter as well as cell growth and death pathways and secondary bile acid synthesis subpathways (ko00121) of lipid metabolism in wild ungulates in winter. Overall, we recommend providing artificial food supplies with high protein and energy contents in deep winter. This preliminary study revealed the relationships between gut microbes of wild red deer and their nutritional status, and more studies should be performed under more rigorous experimental conditions and across species to assess the prevalence of these microbial members in response to nutritional changes in wildlife.
Data availability
The sequencing dataset for gut microbes used in the present study has been submitted to the Sequence Read Archive of the National Center for Biotechnology Information (NCBI) (Bioproject: PRJNA1083038).
Abbreviations
- FN:
-
Faecal nitrogen
- UN:C:
-
Urea nitrogen/creatinine
- LP-LE:
-
Low protein and low energy feeding
- LF:
-
Limited feeding
- SUN:
-
Serum urea nitrogen
- GLU:
-
Glucose
- T3:
-
Triiodothyronine
- T4:
-
Thyroxine
References
-
Zhang, M. H. & Li, Y. Advances in the study of nutritional ecology of ungulate. Chin. J. Wildl. 29(3), 138–146 (2008).
-
Zhang, W. Q. Fine time-scale winter nutritional ecology of deer populations in the lesser Khingan Mountains and Wandashan Mountains, Northeast China. Doctor’s Dissertation (Northeast Forestry University, Harbin, 2014).
-
Wang, L. J., Ma, J. Z., Hong, M. L. & Xiao, X. H. Using Snow urine analysis to assess Roe deer nutritional status in different types of habitats during Winter. Acta Theriol. Sinica. 2, 109–114 (2003).
-
Liu, W. S. Winter diet composition and non-invasive health assessment of feral sika deer. Doctor’s Dissertation (Northeast Forestry University, Harbin, 2012).
-
Delgiudice, G. D., Moen, R. A. & Singer, F. J. Winter nutritional restriction and simulated body condition of Yellowstone elk and bison before and after the fires of 1988. Wildl. Monogr. 147(1), 1–60 (2001).
-
Delgiudice, G. D. & Seal, U. S. Classifying winter undernutrition in deer via serum and urinary urea nitrogen. Wildl. Soc. 16, 27–32 (1988).
-
Hicks, A. et al. Gut microbiomes of wild great apes fluctuate seasonally in response to diet. Nat. Communication. 9, 1786 (2018).
Google Scholar
-
Ley, R. E., Turnbaugh, P. J., Klein, S. & Gordon J. I. Microbial ecology: human gut microbes associated with obesity. Nature. 444, 1022–1023 (2006).
Google Scholar
-
Holmes, Z. C. et al. Short-chain fatty acid production by gut microbiota from children with obesity is linked to bacterial community composition and prebiotic choice. bioRxiv. 11(4), e00914–e00920 (2020).
Google Scholar
-
McGuire, M. K. & McGuire, M. A. Microbiomes and childhood malnutrition: what is the evidence?. Ann. Nutr. Metab. 77(suppl 3), 36–48 (2021).
Google Scholar
-
Wu, W. et al. Undernutrition disrupts cecal microbiota and epithelium interactions, epithelial metabolism, and immune responses in a pregnant sheep model. Microbiol. Spectr. 11(2), 1–17 (2023).
-
Sheng, H. L. The deer in China (East China normal university press, Shanghai, 1992).
-
Niu, Y. Y. et al. Mammal diversity and prey resource of Amur tiger in Heilongjiang Muling Japanese yew national nature reserve. Chin. J. Wildl. 42, 355–362 (2021).
-
Zhong, L. Q. et al. Winter foraging of sympatric red deer and sika deer in northeast China: diet composition, forage selection, bite diameter and browse intensity. J. for. Res. 25(4), 276–284 (2020).
Google Scholar
-
You, Z. et al. Seasonal variations in the composition and diversity of gut microbiota in white-lipped deer (Cervus albirostris). Peerj. 10, e13753 (2022).
Google Scholar
-
Hu, X. et al. High-throughput analysis reveals seasonal variation of the gut microbiota composition within forest musk deer (Moschus berezovskii). Front. Microbiol. 9, 1674 (2018).
Google Scholar
-
Zhang, M. et al. Comparative analysis of gut microbiota changes in Père David’s deer populations in Beijing Milu Park and Shishou, Hubei Province in China. Front. Microbiol. 9, 1258 (2018).
Google Scholar
-
Li, B. et al. Captivity shifts gut microbiota communities in white-lipped deer (Cervus albirostris). Animals. 12, 431 (2022).
Google Scholar
-
Menke, S. et al. Impact of winter enclosures on the gut bacterial microbiota of red deer in the Bavarian Forest National Park. Wildl. Biol. 2019, 1–10 (2019).
-
Wang, X. et al. Comparison of the gut microbiome in red deer (Cervus elaphus) and fallow deer (Dama dama) by high-throughput sequencing of the V3-V4 region of the 16S rRNA gene. Sci. Asia. 45, 515–524 (2019).
Google Scholar
-
Wang, Y. et al. Environmental factors and gut microbiota: toward better conservation of deer species. Front. Microbiol. 14, 1136413 (2023).
Google Scholar
-
Verheyden, H. et al. Faecal nitrogen, an index of diet quality in roe deer Capreolus capreolus? Wildl. Biology. 17(2), 166–175 (2011).
-
Sun, Y. Analysis of winter food composition and nutritional element of red deer in Chifeng Region, Inner Mongolia, China. Master’s Dissertation (Northeast Forestry University, Harbin, 2018).
-
Zhou, L. J. Winter diet of wild red deer (Cervus elaphus xanthopygus) in the forest-grassland ecotone in Inner Mongolia, China. Acta Theriol. Sinica. 42(3), 240–249 (2022).
Google Scholar
-
R Core Team. R: A language and environment for statistical computing (R Foundation for Statistical Computing, Vienna, 2023)https://www.r-project.org/.
-
Carey, H. V. Nutritional ecology of yellow-bellied marmots in the White Mountains of California. Ecography. 8(4), 259–264 (1985).
Google Scholar
-
Saltz, D., White, G. C. & Bartmann, R. M. Assessing animal condition nutrition and stress from urine in snow. Wildl. Soc. Bull. 23(4), 694–704 (1995).
-
White, P. J., Garrott, R. A. & Heisey, D. M. An evaluation of Snow-urine ratios indices of Ungulate Nutritional Status. Can. J. Zool. 75, 1687–1694 (1997).
-
Scott, K. P., Gratz, S. W., Sheridan, P. O., Flint, H. J. & Duncan, S. H. The influence of diet on the gut microbiota. Pharmacol. Res. 69(1), 52–60 (2013).
Google Scholar
-
Guo, J. H. et al. Diet-induced microbial adaptation process of red deer (Cervus elaphus) under different introduced periods. Front. Microbiol. 13, 1033050 (2022).
Google Scholar
-
Guo, J. H., Yuan, Z. A., Zhang, W. Q. & Zhang, M. H. The microbial composition and community diversity of Red Deer (Cervus elaphus xanthopygus) in Winter in Chifeng Region of Inner Mongolia. Chin. J. Wildl. 41(2), 261–271 (2020).
-
Yuan, Z. A. et al. Effects of vegetation type differences induced by human disturbance on the nutrition strategy and gut microbiota of siberian roe deer. Mol. Ecol. 32(10), 2534–2550 (2022).
Google Scholar
-
Yuan, H. Y. A comparative analysis between summer and winter in the intestinal microbiome changes and functional differences of Northeast Sika Deer. Doctor’s Dissertation (Northeast Forestry University, Harbin, 2022).
-
Bao, H. Seasonal adaptation strategy of moose habitat selection, nutrition and gut micro-biome. Doctor’s Dissertation (Northeast Forestry University, Harbin, 2019).
-
Kameshwar, A. K. S. & Qin, W. S. Recent developments in using advanced sequencing technologies for the genomic studies of lignin and cellulose degrading microorganisms. Int. J. Biol. Sci. 12(2), 156 (2016).
Google Scholar
-
Jami, E., Israel, A., Kotser, A. & Mizrahi, I. Exploring the bovine rumen bacterial community from birth to adulthood. ISME J. 7, 1069–1079 (2017).
-
Chevalier, C. et al. Gut microbiota orchestrates energy homeostasis during cold. Cells. 163, 1360–1374 (2015).
Google Scholar
-
Su, R., Dalai, M., Luvsantseren, B., Chimedtseren, C. & Hasi, S. Comparative study of the function and structure of the gut microbiota in siberian musk deer and forest musk deer. Appl. Microbiol. Biotechnol. 106, 6799–6817 (2022).
Google Scholar
-
Aristilde, L. Metabolite labelling reveals hierarchies in Clostridium acetobutylicum that selectively channel carbons from sugar mixtures towards biofuel precursors. Microb. Biotechnol. 10, 162–174 (2017).
Google Scholar
-
Robert, C. & Bernalier-Donadille, A. The cellulolytic microflora of the human colon: evidence of microcrystalline cellulose-degrading bacteria in methane-excreting subjects. FEMS Microbiol. Ecol. 46(1), 81–89 (2003).
Google Scholar
-
Turnbaugh, P. J. et al. An obesity-associated gut microbiome with increased capacity for energy harvest. Nature. 444(7122), 1027–1031 (2006).
Google Scholar
-
Balamurugan, R., George, G., Kabeerdoss, J. & Aarthy, M. S. J. H. quantitative differences in intestinal Faecalibacterium prausnitzii in obese Indian children. Br. J. Nutr. 103(3), 335–338 (2010).
Google Scholar
-
Furet, J. P. et al. Differential adaptation of human gut microbiota to bariatric surgery-induced weight loss links with metabolic and low-grade inflammation markers. Diabetes. 59(12), 3049–3057 (2010).
Google Scholar
-
Smith, E. A. & Macfarlane, G. T. Enumeration of amino acid fermenting bacteria in the human large intestine: effects of pH and starch on peptide metabolism and dissimilation of amino acids. FEMS Microbiol. Ecol. 25, 355–368 (1998).
Google Scholar
-
Smith, E. A. & Macfarlane, G. T. Enumeration of human colonic bacteria producing phenolic and indolic compounds: effects of pH, carbohydrate availability and retention time on dissimilatory aromatic amino acid metabolism. J. Appl. Bacteriol. 81, 288–302 (1996).
Google Scholar
-
Kohl, K. D. & Carey, H. V. A place for host-microbe symbiosis in the comparative physiologist’s toolbox. J. Exp. Biol. 219(Pt 22), 3496–3504 (2016).
Google Scholar
-
Ullrey, D. E. et al. Digestibility of Cedar and Balsam Fir browse for the White-tailed deer. J. Wildl. Manage. 32, 162–171 (1968).
-
Bahnak, B. R., Holiand, J. C., Verme, L. J. & Ozoga, J. J. Seasonal and nutritional influences on growth hormone and thyroid activity in white-tailed deer. J. Wildl. Manage. 45(1), 140–147 (1981).
Google Scholar
-
Brown, R. D., Hellgren, E. C., Abbott, M., Ruthven, D. C. III & Bingham, R. L. Effects of dietary energy and protein restriction on nutritional indices of female white-tailed deer. J. Wildl. Manage. 59(3), 595–609 (1995).
-
Zhang, K. et al. Taxonomic and functional adaption of the gastrointestinal microbiome of goats kept at high altitude (4800 m) under intensive or extensive rearing conditions. FEMS Microbiol. Ecol. 97(3), fiab009 (2021).
Google Scholar
-
Monira, S. et al. Gut microbiota of healthy and malnourished children in Bangladesh. Front. Microbiol. 2, 228 (2011).
Google Scholar
-
Zenner, C. et al. Early-life immune system maturation in chickens using a synthetic community of cultured gut bacteria. mSystems. 6(3), e01300–e01320 (2021).
Google Scholar
-
Kübeck, R. et al. Dietary fat and gut microbiota interactions determine diet-induced obesity in mice. Mol. Metabolism. 5, 1162–1174 (2016).
-
Lefebvre, P. et al. Role of bile acids and bile acid receptors in metabolic regulation. Physiol. Rev. 89(1), 147–191 (2009).
Google Scholar
-
Kanehisa, M. & Goto, S. K. E. G. G. Kyoto encyclopedia of genes and genomes. Nucleic Acids Res. 28, 27–30 (2000).
Google Scholar
-
Collins, S. L., Stine, J. G., Bisanz, J. E., Okafor, C. D. & Patterson, A. D. Bile acids and the gut microbiota: metabolic interactions and impacts on disease. Nat. Rev. Microbiol. 21(4), 236–247 (2023).
Google Scholar
-
De Vallim, A., Tarling, T. O. & Ed-Wards, E. J. Pleiotropic roles of bile acids in metabolism. Cell Metabol. 17(5), 657–669 (2013).
-
Xue, Y. F., Guo, C. Z., Hu, F., Zhu, W. Y. & Mao, S. Y. Undernutrition-induced lipid metabolism disorder triggers oxidative stress in maternal and fetal livers using a model of pregnant sheep. FASEB J. 34(5), 6508–6520 (2020).
Google Scholar
Acknowledgements
We would like to thank the leaders and staff of the Gaogesitai National Nature Reserve for their strong support and assistance in this research, and like to thank Professor C. Zhang for his valuable advice on the manuscript writing.
Plants collection approval statements
The collection of plants (including Quercus mongolica, Ulmus pumila, Salix rosmarinifolia, Ostryopsis davidiana and Prunus sibirica) was permitted by the Reserve Management Authority and the local Forestry Bureau, and the process was in accordance with the ARRIVE guidelines 2.0.
Funding
This research was funded by the Opening Research Projects for the Think Tanks of Heilongjiang Provincial Universities (ZKKF2022179); National Key Research and Development Program of China: Migration and diffusion mechanism of wild animals and population control technology (2023YFF1305000); the National Natural Science Foundation of China, NSFC (32071512); National Key Fundamental Research Funds for the Central Universities, China (2572023AW20; 2572020BE02); the Guizhou Forestry Administration Scientific Research Project (QLKH [2023] 11); and the Reward and Subsidy Fund Project of Guizhou Education University, Ministry of Science and Technology of the People’s Republic of China and National Natural Science Foundation of China (2023GZJB005).
Author information
Authors and Affiliations
Contributions
Jinhao Guo conceived the ideas and designed the methodology; Jinhao Guo and Zheng Li collected the data; Jinhao Guo, Zheng Li, and Xinxin Liu analysed the data; Jinhao Guo, Zheng Li, Xinxin Liu, and Yongchao Jin led the writing of the manuscript; Yongchao Jin, Yue Sun, Ziao Yuan, Weiqi Zhang participated in the main revision of the manuscript; Jialong Wang and Minghai Zhang participated in the revision of the manuscript and provided research funds. All authors contributed critically to the drafts and gave final approval for publication.
Corresponding authors
Ethics declarations
Ethics approval
All experiments and methods followed the principles of the Ethical Committee for Experimental Animal Welfare of the Northeast Forestry University, and were approved by Conservation Authority and local Forestry Bureau. All methods performed in this study were strictly in compliance with the Wild Animals Conservation Laws of China. The study followed the ARRIVE guidelines 2.0.
Consent for publication
Not applicable.
Competing interests
The authors declare no competing interests.
Additional information
Publisher’s note
Springer Nature remains neutral with regard to jurisdictional claims in published maps and institutional affiliations.
Supplementary Information
Supplementary Material 1.
Supplementary Material 2.
Supplementary Material 3.
Supplementary Material 4.
Rights and permissions
Open Access This article is licensed under a Creative Commons Attribution-NonCommercial-NoDerivatives 4.0 International License, which permits any non-commercial use, sharing, distribution and reproduction in any medium or format, as long as you give appropriate credit to the original author(s) and the source, provide a link to the Creative Commons licence, and indicate if you modified the licensed material. You do not have permission under this licence to share adapted material derived from this article or parts of it. The images or other third party material in this article are included in the article’s Creative Commons licence, unless indicated otherwise in a credit line to the material. If material is not included in the article’s Creative Commons licence and your intended use is not permitted by statutory regulation or exceeds the permitted use, you will need to obtain permission directly from the copyright holder. To view a copy of this licence, visit http://creativecommons.org/licenses/by-nc-nd/4.0/.
Reprints and permissions
About this article
Cite this article
Guo, J., Li, Z., Liu, X. et al. Response of the gut microbiota to changes in the nutritional status of red deer during winter.
Sci Rep 14, 24961 (2024). https://doi.org/10.1038/s41598-024-76142-1
-
Received: 15 May 2024
-
Accepted: 10 October 2024
-
Published: 23 October 2024
-
DOI: https://doi.org/10.1038/s41598-024-76142-1
Keywords
- Ungulates
- Nutritional status
- Population conservation
- Gut microbes
- Metagenomic sequencing