Abstract
Frontier scientific discoveries increasingly rely on big-science research infrastructures, such as supercolliders, synchrotron light sources, and space telescopes, whose construction and operation involve intensive international collaboration. This collaborative nature, however, presents a challenge in balancing national interests with the common good, particularly given the substantial fiscal investments involved. This study investigates the effects of one of China’s prominent big-science infrastructures, the Shanghai Synchrotron Radiation Facility (SSRF), on the country’s production of science, differentiating between national and international scientific endeavors. Employing a rigorous difference-in-differences (DID) methodology, it evaluates SSRF’s effects on discipline-level production of publications by domestic researchers and those involving international collaboration. The empirical findings reveal compelling evidence that SSRF has significantly enhanced the production of high-impact national and international publications for disciplines reliant on the facility for experiments, particularly increasing the percentage of high-impact national publications. The findings also underscore the dual role of big-science infrastructures in fostering international research collaboration while simultaneously enhancing nations’ domestic scientific development and competitiveness. Additionally, the study provides valuable insights for research assessment and science policy discussions.
Similar content being viewed by others
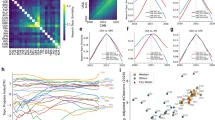
Quantifying progress in research topics across nations
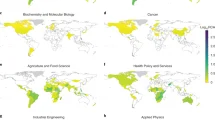
The latent structure of global scientific development
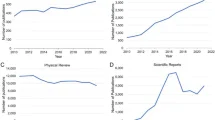
The influence of Chinese scholars on global research
Introduction
Since the mid-twentieth century, a trend has prevailed in the global scientific enterprise, where big-science research infrastructures have increasingly contributed to discoveries at the frontier of science (Weinberg, 1961; Price, 1963, 1986; Hallonsten, 2016; Cramer et al., 2020). The advanced experimental opportunities offered by supercolliders, synchrotron light sources, space telescopes, and others have enabled scientific breakthroughs in various fields, including physics, chemistry, materials science, and life sciences. For example, hosting big-science research infrastructures, U.S. national laboratories, and the European Organization for Nuclear Research (CERN) have achieved breakthroughs, leading to quite a number of Nobel Prizes.
Furthermore, scientific discoveries obtained from these machines and technologies used in constructing and operating them have generated spillover in terms of innovation and applications as well as raising the profiles of nations hosting them. Consequently, following the trajectory demonstrated by developed countries, emerging countries have jumped on the bandwagons by allocating huge amounts of resources to developing these facilities, not only aiming to raise their profile but also hoping to eventually reap returns to the investment and drive social welfare (Alberts, 2012; Beck and Charitos, 2021; Yang et al., 2023; Yang et al., 2024). In the process, scientists in emerging countries shift their activities from learning by using these facilities to creating and contributing new knowledge and eventually moving to the global frontier of science.
Meanwhile, the construction and operation of big-science infrastructures typically involve extensive international collaborations (Georghiou, 1998; Crease, 1999; Hand, 2010). Examples range from the Manhattan Project to the utilization of the Large Hadron Collider (LHC) and Laser Interferometer Gravitational-Wave Observatory (LIGO). However, this collaborative nature presents the challenge of “balancing between national interests and the common good” (Hallonsten, 2014, p. 31). From a scientific perspective, attracting top scientists from around the world to conduct experiments is crucial for the performance of big-science research infrastructures, particularly for technologically peripheral or semi-peripheral nations (Traweek, 1992). Nevertheless, from a political standpoint, financed primarily by taxpayers, these infrastructures are expected to align with national agendas that prioritize enhancing domestic scientific development and technological competitiveness (Jang and Ko, 2019).
Drawing from the theory on technology catch-up (Nelson and Phelps, 1966; Abramovitz, 1986), we argue that there exist two types of scientific innovation: one is indigenous innovation produced by domestic scientists, and the other is collaborative innovation conducted through collaboration with international scientists. For latecomer countries, international scientific collaboration can help scientists access cutting-edge knowledge and obtain research resources and information advantages that are not available domestically, which is an important way to enhance national basic research capabilities (Hwang, 2008). As the gap between latecomer and leading countries gradually narrows, latecomer countries’ mode of scientific progress may gradually shift from the collaborative to the indigenous one (Acemoglu et al., 2006), during which big-science infrastructures can play important roles.
Against these backdrops, we investigate the effect of a major big-science infrastructure in China, which as a rising scientific power, has enlarged the scale and scope of investing in R&D, including big-science facilities (Crow, 2024). The focal infrastructure is the Shanghai Synchrotron Radiation Facility (SSRF), the first and only 3rd generation synchrotron light source in Mainland China. It has been operating steadily since 2009, attracting over 26,000 domestic and foreign scientists who have conducted cutting-edge experiments there. When assessing the impact of SSRF, we are particularly interested in distinguishing between national science produced by domestic researchers (i.e., through indigenous innovation) and international science involving cross-border research collaboration (i.e., through collaborative innovation).
The analytical challenge in studying the effects of a big-science infrastructure on the production of science lies in the endogeneity problem of the infrastructure. Existing literature has mostly relied on bibliometric methods to analyze the direct outputs of the infrastructures, such as scientific publications and patents (Zhang et al., 2011; Hallonsten, 2013; Heidler and Hallonsten, 2015; Carrazza et al., 2016). However, this approach has limitations in capturing the indirect impact of the infrastructures. Typically, the direct outputs of the big-science infrastructures are likely to further enhance the scientific research in related disciplines, as science tends to progress cumulatively (Furman and Stern, 2011). Additionally, visible scientific outputs have the potential to attract more talent and funding, thus further accelerating the development of related disciplines.
In contrast to the aforementioned bibliometric studies, we adopt a difference-in-differences (DID) econometric method to estimate the effect of SSRF on the discipline-level production of scientific knowledge. A recent study (Yang et al., 2024) also uses the DID design to examine the impact of big-science research infrastructures on regional knowledge innovation, taking China’s National Supercomputing Center (NSC) as a case, but their analysis is performed at the city level. Our study uniquely delves into the differentiation between national science produced by domestic researchers and international science involving cross-border research collaboration at the discipline level.
Our identification strategy hinges on the fact that only certain scientific disciplines rely on synchrotron light for conducting experiments and are, therefore, affected by the construction of SSRF. These disciplines include various subfields in physics, chemistry, materials science, and biomedical science, which can be regarded as our “treatment group.” On the other hand, the development of many other scientific subfields, such as mechanics, meteorology, and clinical medicine, does not depend on the presence of synchrotron light. These disciplines can serve as our “control group.”
For instance, in the realm of medical science, specific disciplines like clinical neurology, endocrinology, metabolism, hematology, and imaging science stand to gain significantly from the establishment of SSRF as it facilitates researchers to undertake cutting-edge experiments, thereby accelerating advancements in these fields. Conversely, other disciplines, including anesthesiology, clinical neurology, allergy, and nutrition, seldom utilize synchrotron light for their research. The disparity in the rate of development between these two groups post-SSRF establishment can, therefore, be attributed to the facility’s influence, conditioning on a “parallel trend” observed prior to the facility’s inception.
Our analysis is performed on a dataset of 1.75 million scientific publications indexed by the Web of Science, each with at least one author based in China, spanning the period from 1998 to 2015. Using the opening of SSRF in 2009 as a quasi-natural experiment, our DID estimations reveal that SSRF has significantly enhanced the percentage of high-impact national publications within the total number of discipline-level publications. Compared to the control-group disciplines that do not use synchrotron light for experiments, the treatment-group disciplines experienced a 12.4% increase in their percentage of high-impact national publications after SSRF was put into use. However, SSRF’s effect on the percentage of high-impact international publications is not statistically significant. When considering the overall impact of publications, we observe a noticeable improvement in the average impact factor (IF) of journals in which the treatment-group’s publications, both national and international, appeared, compared to publications of the control-group disciplines.
Estimating the dynamic effects of SSRF at different time points confirms that the treatment and control groups satisfied the “conditional parallel trend” condition before 2009. Furthermore, our results remain robust when we perform various robustness tests, such as a falsification test with randomized treatment and control groups and a test with placebo treatments prior to the real SSRF shock. Overall, our results suggest that the establishment of SSRF has significantly enhanced the production of high-impact national and international publications, with a particularly notable effect on increasing the percentage of high-impact national publications.
The study contributes to several streams of the literature in sociology, economics, and politics of science, including knowledge production, research assessment, and science policy. First, we are interested in how big-science infrastructures influence knowledge production within national borders and through international collaboration. We highlight the significance of SSRF and likely similar facilities in fostering high-impact national publications as well as facilitating China’s pursuit of the global scientific frontier. The international experience suggests that scientists in latecomer countries often start by learning from and collaborating with their counterparts in advanced countries (Hwang, 2008; Kim, 2006). Gradually, while continuing to leverage the international scientific network, scientists in developing countries also engage in producing knowledge through indigenous efforts (Smith et al., 2011). This is especially the case for China, which has witnessed those pursuing study and research opportunities at the international frontiers of science nurturing new opportunities for research and learning upon returning and engaging in the creation of science at home (Cao et al., 2020). Big-science infrastructures such as SSRF provide a fruitful ground for examining such effects on knowledge production involving the efforts of national and international scientists.
Second, this study makes efforts to respond to the increasing concerns about the payoff of investment in R&D, scientific productivity, and research assessment. Indeed, government needs to be accountable for such investment, and scientists need to be productive to fulfill their social contracts (Guston, 2000). In this case, research assessment exercises have proliferated worldwide to evaluate the effectiveness, efficiency and spillovers of public funding programs including big-science programs, or the measurable returns on investment in big-science infrastructures, considering not only scientific publications but also industrial R&D, scientific networks, and broader socioeconomic values (e.g., ESFRI, 2018; Heidler and Hallonsten, 2015; Helmers and Overman, 2017; OECD, 2019). Focusing on the scientific impact of typical big-science research infrastructure and differing from existing descriptive and bibliometric studies of specific research infrastructures (e.g., Hallonsten, 2013, 2014; Martin and Irvine, 1981, 1983; Zhang et al., 2011), our DID econometric design partly overcomes the endogeneity issue of big-science infrastructures and is able to infer the impact of SSRF on discipline-level science production.
Third, we expect our research to generate implications for science policy on the investment of R&D and especially basic research. As big-science research infrastructures are mostly financed by taxpayers and resources are always scarce, the government needs to consider the tradeoff between investment in such facilities and in other areas that may have more immediate payoffs (Stephan, 2012; Weinberg, 1961) as well as that between funding small- vs. big-science projects (Fortin and Currie, 2013; Price, 1963, 1986). That is, it is in the government’s strategic interests to take a multifaceted research approach that encompasses curiosity-driven projects, mission-oriented programs, and large-scale scientific endeavors. In the past few decades, there have been heated debates on whether governments should shift funding toward smaller-scale, individual-investigator-initiated research projects (Alberts, 2012; Berg, 2017; Wei et al., 2021). Big-science projects, such as the Superconductor Supercollider in the United States, were even terminated after partial construction (Kevles, 1997). It is scientific as well as political to decide on which and where to invest. This is especially the case for a latecomer country in science, such as China, where science policymakers have to consider whether the prioritization in one area would be at the expense of others. Our study thus contributes to policy discussions with new empirical evidence on the scientific effects of a prominent big-science infrastructure in China, which at least represents one type of payoff from an academic perspective.
Data and empirical methodologies
Data
Our initial sample included 1,908,610 English-language journal publications with at least one author based in China, including Hong Kong, Macau, and Taiwan, from 1970 to 2015. The data were extracted from the Web of Science, which, as one of the world’s largest databases of academic publications, contains detailed information about each paper, including its title, journal, journal ISSN, language, keywords, abstract, author names, author institutions, reprint author, funding, references, publishing date, scientific discipline(s), and the number of citations (see the supplementary information for an example of the publication data).
Given that we are interested in the effect of SSRF on disciplinary scientific development, we excluded 55,584 publications from 76 multi-disciplinary journals, such as Nature and Science, to ensure our results are not driven by Chinese scientists possibly publishing more extensively in these journals. We then merged the publication data with data on the journal IF from the Journal Citation Reports, also maintained by the Web of Science. Since 1998 was the first year when systematic journal IF statistics were available in our sample, our final sample consisted of 1,750,298 publications from 1998 to 2015.
Empirical design
Synchrotron radiation light possesses a range of unique and superior properties for scientists to see previously unseen phenomena and processes, bringing revolutionary advancement to science and human civilization. Our conversations with scientists conducting experiments at SSRF confirmed its indispensability: They regarded SSRF as crucial to their research and reported that before its existence, they either had to use similar synchrotron facilities abroad, for example, the Super Photon Ring-8 GeV (SPring-8) in Japan, or they could not pursue their specific lines of research. Hence, we expect the establishment of SSRF to substantially lower the barrier for Chinese domestic scientists to conduct novel and cutting-edge experiments that lead to scientific advancement.
Empirically, the main challenge in establishing a causal link between the operation of SSRF and the generation of new scientific knowledge is the potential endogeneity of SSRF. Focusing the analysis on scientists who have conducted experiments at SSRF and their publications could introduce various selection bias and reverse causality issues. For example, the scientists being selected to use SSRF may have superior research performance compared to those not chosen,
In contrast, we exploit the fact that due to the physical properties of synchrotron radiation light and its potential for enabling certain scientific experiments, some disciplines would directly benefit from the construction of domestic synchrotron radiation facilities like SSRF, while the development of many other disciplines stays unimpacted. This heterogeneity in SSRF’s relevancy to different disciplines allows us to use the following DID model for estimating the effect of SSRF on the discipline-level production of scientific publications:
y i,t are measures of the research output of discipline i in year t and include: (1) High IF publication percent, which is the percentage of publications that appeared in journals with an IF > 10 to total publications; and (2) Mean IF, which is the average IF of the journals in which the discipline’s publications appeared. To ensure that our main results are not driven by the specific cutoff of IF > 10, we have used alternative cutoffs such as IF > 5 to define High IF publication, and our results remain robust. We further differentiate two types of publications—national publications that are authored by China-based researchers and international publications that involve cross-border research collaboration—to study the differential effects of SSRF on these two distinct forms of science production.
Treatment i is a dummy variable that equals 1 if discipline i can utilize SSRF to conduct experiments, and it equals 0 if otherwise. The Web of Science Core Collection categorizes all journal articles into 252 scientific disciplines. In assigning publications to treatment and control groups, we have excluded all publications in social science and humanities disciplines. Our empirical strategy thus explores cross-disciplinary heterogeneity within the domain of natural science research. To accurately identify the treatment-group disciplines that have been impacted by the operation of SSRF, we have employed a combined approach of literature analysis and expert rating by SSRF’s managers and beamline scientists.
Specifically, we first contacted SSRF for the list of publications coming directly from research with it. The disciplinary domains indicated by these papers were employed to preliminarily delineate the affected disciplines. The expert rating stage involved the active participation of key stakeholders, including two SSRF user management staff and three beamline scientists, who provided valuable insights to verify and update the scope of the affected disciplines. The detailed procedures are presented in the supplementary information. As a result, 117 narrowly defined scientific disciplines whose scientists can conduct experiments at SSRF are considered as our treatment group. The remaining 96 disciplines constitute the control group.
After t is a dummy variable that equals 1 after the year 2009, when SSRF was first opened to users, and it equals 0 before or in 2009. Controli,t are control variables that include NSFC funding, which is the logarithm of the total amount of funding each broad scientific field receives from the National Natural Science Foundation of China (NSFC) each year. The data was sourced from the official website of NSFCFootnote 1. NSFC supports eight broad scientific fields, including Mathematical and Physical Sciences, Chemical Sciences, Life Sciences, Earth Sciences, Engineering and Material Sciences, Information Sciences, Management Sciences, and Medical Sciences. We manually matched specific Web of Science (WoS) disciplines to these fields. As noted, social science and humanities disciplines have been excluded from our analysis, so the 213 WoS disciplines used in the analysis are mostly in science, technology, and engineering. We first conducted the mapping independently. In cases of disagreement, we collectively deliberated to reach a consensus. Consequently, 207 out of the 213 WoS disciplines were successfully mapped to a NSFC scientific field, with the exception of those related to psychology, archeology, and demography.
We have further controlled for Graduate student, which is the logarithm of the number of postgraduate students (including Master’s and PhD) in each broad scientific field in each year. The data was obtained from China Statistic Yearbook on Science and Technology. Graduate students were categorized into 13 fields, including science, engineering, medicine, agriculture, philosophy, economics, management, education, law, literature, history, military, and art. We applied the same manual mapping procedure to align the WoS disciplines with these fields. As a result, almost all of the WoS disciplines in our analysis were mapped to a given field, with the exception of “family studies” and “planning & development.”
Finally, we controlled for Overseas returnee, which is the logarithm of the total number of returnees to China that received education or conducted research in foreign countries in each year. This data was obtained from the website of the Ministry of Education of China. It is aggregated, without differentiating specific disciplines.
The model also includes discipline fixed effects δ i and year fixed effects δ t. It is worth noting that although governmental support to expedite the development of targeted areas will affect our dependent variables, it is unlikely that governmental support only applies to specific treatment-group disciplines. Even specific targeted areas like nanotechnology, quantum computing, and bioinformatics, which have been increasingly emphasized by the Chinese government in recent years, are likely to impact both the treatment- and control-group disciplines in our sample. Furthermore, even external factors may have disproportionately impacted the treatment- and control-group disciplines. As long as the degree of disparity does not vary with time, this difference should have been controlled for given that we included δ i in our model.
Our interested coefficient is β1 of the interaction term Treatment i*After t, which captures the effect of SSRF on the science production of affected disciplines compared to disciplines that do not utilize SSRF, after the facility’s opening in 2009. Table 1 presents the summary statistics of our main variables.
Results
Before formally estimating the DID model, we present the data pattern through the aggregation of raw data for the treatment- and control-group disciplines. Figure 1 illustrates the time trend of the High IF publication percent for both groups from 1998 to 2015, considering both national publications (Panel A) and international publications (Panel B). It is evident that the two groups exhibit similar pre-trends prior to the operation of SSRF in 2009, as the percentages of high-impact national and international publications are comparable. However, the disparity between the two groups becomes more pronounced after 2009, particularly when zooming in on national publications. Similarly, Fig. 2 displays the time trend of the Mean IF of publications for the treatment-group and control-group disciplines. Prior to 2009, the two groups also demonstrate relatively parallel pre-trends, yet the differences between them are amplified after 2009, both for national and international publications.
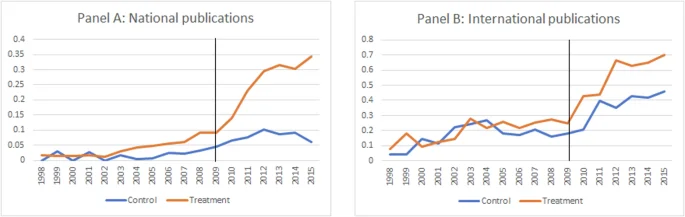
The figure plots the time trend of concerned variables for the treatment group (scientific disciplines that utilize SSRF for experimentation) and the control group (scientific disciplines that are not affected by the operation of SSRF) by taking the average of the respective raw data (High IF publication percentage). Panel A is concerned with national publications produced by domestic researchers and Panel B is concerned with international publications involving cross-border research collaboration. It shows that the two groups had similar “pre-trends” before the opening of SSRF in 2009. However, the difference between the two groups becomes more substantial after 2009, especially when national publications are concerned.
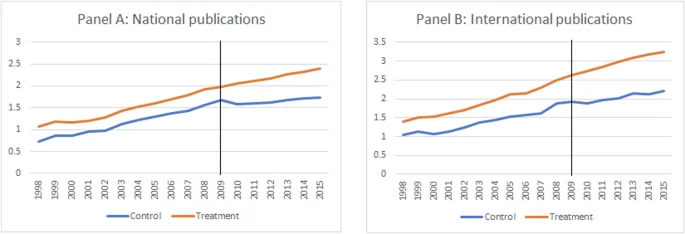
The figure plots the time trend of concerned variables for the treatment group (scientific disciplines that utilize SSRF for experimentation) and the control group (scientific disciplines that are not affected by the operation of SSRF) by taking the average of the respective raw data (Mean IF). Panel A is concerned with national publications produced by domestic researchers and Panel B is concerned with international publications involving cross-border research collaboration. It shows that the two groups had similar “pre-trends” before the opening of SSRF in 2009. However, the difference between the two groups becomes more substantial after 2009.
We quantified the abovementioned effects by estimating the DID model in equation (1) and present the estimation results in Table 2. Models (1) and (2) examine the effect of SSRF on High IF publication percentage. Model (1) shows that within our sample period from 1998 to 2015, SSRF had been associated with a 12.4% increase in the percentage of high-IF national publications for the treatment-group disciplines. However, according to Model (2), SSRF’s effect on the percentage of high-IF international publication is insignificant. Delving into SSRF’s influence on the overall IF of discipline-level publications, Model (3) shows that SSRF had been associated with a 0.143 increase in the Mean IF of national publications for the treatment-group disciplines, corresponding to a 9.47-percent increase over the sample mean, and Model (4) reveals that SSRF had been associated with a 0.334 increase in the Mean IF of international publications for the treatment-group disciplines, or a 17.67-percent increase over the sample mean. That is, the impact of SSRF is more significantly reflected in improving the quality of internationally collaborated publications than in that of domestic publications.
The validity of our baseline DID regression results depends on whether the treatment- and control-group disciplines had similar trends before SSRF’s opening in 2009. Although the data pattern shown in Figs. 1 and 2 have to some extent, validated this “parallel trend,” to further verify this assumption, we replaced the Treatment i*After t in equation (1) with a set of dummy variables, denoted as Treatment i*After t,k, where k indicates the kth year before or after the operation of SSRF in 2009. We then test whether the coefficient β1, k is statistically equal to 0 for k < 0 and statistically larger than 0 for k > 0. The results of these tests are illustrated in Figs. 3 and 4, in which the horizontal axis measures the number of years before or after 2009, and the vertical axis represents the estimated β1, k in regressions in which the dependent variable is either the High IF publication percent (Fig. 3) or the Mean IF (Fig. 4).
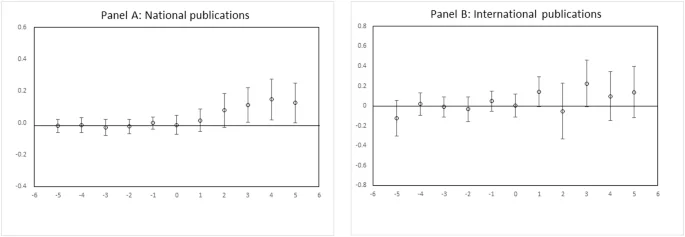
The horizontal axis represents the time relative to the reference year, which is 2009, when SSRF was first put into use. “−5” is the fifth year prior to the reference year, and “5” is the fifth year after the reference year. The dots represent the estimated coefficients for the effect of SSRF on the treatment group (scientific disciplines that utilize SSRF for experimentation) compared to the control group (scientific disciplines that are not affected by the operation of SSRF) in each corresponding year. The solid line shows the 90 percent confidence intervals and standard errors are clustered at the discipline level. Panel A is concerned with national publications produced by domestic researchers and Panel B is concerned with international publications involving cross-border research collaboration.
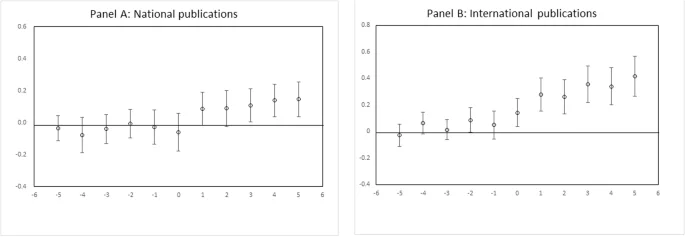
The horizontal axis represents the time relative to the reference year, which is 2009, when SSRF was first put into service. “5” is the fifth year prior to the reference year, and ‘5’ is the fifth year after the reference year. The dots represent the estimated coefficients for the effect of SSRF on the treatment group (scientific disciplines that utilize SSRF for experimentation) compared to the control group (scientific disciplines that are not affected by the operation of SSRF) in the corresponding year. The solid line shows the 90 percent confidence intervals and standard errors are clustered at the discipline level. Panel A is concerned with national publications produced by domestic researchers and Panel B is concerned with international publications involving cross-border research collaboration.
Consistent with our main findings in Table 2, the estimated coefficients β1, k for national publications, shown in Fig. 3 Panel (A), are close to zero and insignificant for k < 0. Only in the period with k > 2, does the estimated β1, k become positive and significant. In Panel (B), when international publications are concerned, the estimated β1, k are not significantly different from 0 for any choice of k, confirming the insignificant result we find in the baseline analysis. In Fig. 4, both panels show similar trends for the treatment- and control-group disciplines: the estimated coefficients β1, k are close to zero and almost all insignificant for k < 0, confirming that the estimates in Table 2 are unlikely affected by unobserved omitted variables. Only after SSRF’s opening in 2009 do the estimated coefficients β1,k (k > 0) become significantly larger than 0 for both national publications (Panel (A)) and international publications (Panel (B)).
These findings suggest that investment in big-science infrastructures can effectively enhance the overall production of high-impact science. Moreover, such an effect is more prominent for national science compared to international science in our case, given that only the percentage of high-impact national publications is significantly increased by the operation of SSRF.
Robustness tests and additional analyses
To provide further evidence that our main results are not driven by unobservable characteristic of the treatment-group disciplines, we conducted a falsification test (Oster, 2019; Holt and Sullivan, 2023). Specifically, we randomized the probability of any discipline that falls into the treatment group. If some underlying characteristics of the disciplines have caused our main findings from the DID estimations, there is some probability that estimations using the randomized treatment group may also show significant effects of SSRF on the production of science. In contrast, if the main results are indeed caused by SSRF through providing cutting-edge experimental resources to related disciplines, we expect to find insignificant results if the treatment-group disciplines are “placebos” through randomization.
To do so, we used a uniform distribution to generate 1000 randomized placebo treatment groups. We then estimated our DID model in equation (1) 1000 times using the corresponding randomized treatment- and control-group disciplines. As shown in Table 3, when either High IF publication percent or Mean IF is concerned, the coefficients based on the randomized data are close to zero and differ statistically from the coefficients estimated using the actual data. Thus, these results provide additional evidence to rule out the alternative explanation that unobservable discipline characteristics would have driven our results.
Furthermore, to verify that the effect of SSRF only takes place after 2009, we conducted a robustness test with placebo treatments prior to the real SSRF shock. Specifically, we created a set of placebo shocks that happened before 2009. For instance, “1 year” indicates that the placebo shock dummy equals 1 for the year prior to the real opening of SSRF in 2009, and “5 years” indicates that the placebo shock dummy equals 1 for the five years prior to the real opening of SSRF. We then re-estimated our baseline model by replacing the original After t dummy, which equals 1 after 2009, with the placebo dummy. The results are reported in Table 4. The estimated β1 is almost all insignificant or negative, further confirming that the positive effect of SSRF indeed has occurred since 2009.
Finally, given that it takes time to publish one’s research, particularly high-impact one, we need to consider the lag of the effect of SSRF. As a robustness check, we re-estimated the baseline model by lagging all the explanatory and control variables by one year to reflect potential delays in generating impacts. As shown in Table 5, the results are highly consistent with our baseline results: compared to the control-group disciplines that do not use synchrotron light for experiments, the treatment-group disciplines experienced a 14.4% increase in their percentage of high-impact national publications after SSRF was put into use. We also observed a noticeable improvement in the average IF of publications in the treatment-group disciplines, both for national and international publications, compared to the control-group disciplines. The economic magnitude of the effects is also similar to that in the baseline estimations.
To examine whether the effect of SSRF varies across broader scientific fields, we grouped the 117 treatment-group disciplines into six scientific fields: physics, chemistry, materials science, engineering, biomedical science, and environmental science. We then re-estimated our baseline DID model for six times with each field being the treatment group. The control group is the same as that in the baseline estimation, which includes the 96 scientific disciplines that do not use synchrotron facility for experiments. The results are summarized in Table 6, focusing on the overall IF of publications (Mean IF). When international publications are concerned, SSRF has a significantly positive effect for all the fields. Yet its effect on national publications becomes insignificant for the fields of physics, biomedical science and environmental science. This may suggest that international collaboration in using SSRF is more essential for these particular fields to generate high-IF scientific outputs.
Notably, this finding is consistent with a bibliometric analysis we have conducted based on the publications coming directly from research with SSRF. Specifically, among the top 20 disciplines ranked by the number of publications, there is a high level of heterogeneity in terms of the percentage of publications involving international collaboration. Fields with the highest level of international collaboration include optics, environmental science, condensed matter physics, environmental engineering, and cell biology, which all fall under the broader categories of physics, biomedical science, and environmental science fields. This widespread international collaboration may partially explain why the effect of SSRF on the national publications of these fields is not significant.
Discussion
The utilization of big-science infrastructures that came into being during the nuclear era did not cease at the end of these periods. Rather, the past half century has witnessed a boom of such facilities worldwide, first in the United States, European countries, Japan, and now in developing countries such as China, Brazil, and India, as these facilities continuously generate scientific breakthroughs and facilitate the development of cutting-edge technologies. These infrastructures are especially important for latecomers’ catchup with or even surpassing developed countries in certain scientific disciplines (Traweek, 1992; Wei et al., 2021).
Using a novel DID design, we investigated the effect of SSRF, a major big-science infrastructure in China, on discipline-level science production. Our analysis shows that SSRF can significantly enhance the production of high-impact scientific publications in disciplines that utilize the facility, particularly for national science conducted by domestic researchers. Furthermore, the operation of the infrastructure has increased the overall impact of both national and international scientific publications for affected disciplines. The findings contribute to a growing body of literature on the impacts of government R&D funding in general (Howell, 2017; Li et al., 2017; Fleming et al., 2019) and the impacts of such funding for big-science projects in particular (Beck and Charitos, 2021; Hallonsten, 2013; Martin and Irvine, 1983; Florio and Sirtori, 2016; Helmers and Overman, 2017).
The study also generates implications for science policy making regarding internationalization of science and government investment in R&D. For the former, while endeavoring to strengthen their indigenous capability for achieving breakthroughs in science and translating them into innovation, nations still need to “walk on two legs” to leverage international collaboration for approaching frontiers of research. China’s rise to a formidable position in science and innovation is a testimony to the value of such a strategy (Appelbaum et al., 2018). Meanwhile, governments need to invest in a portfolio of research, from curiosity-driven, individual-investigator-initiated projects, mission-oriented R&D programs, to big-science research infrastructures as overemphasis of one aspect at the expense of others may not be in the nations’ best interests and may not result in best results.
However, several challenging questions arising from our discoveries require further research. Above all, our DID estimations still suffer from several limitations. For instance, the estimates would be biased if other shocks disproportionately impact the treatment- and control-group disciplines after 2009. Furthermore, although we explored the heterogeneous effect of SSRF across broad scientific fields, our estimates cannot capture the heterogeneity of treatment effects across narrower disciplines. Given that the establishment of the SSRF represents a one-time shock, our model can only estimate the average treatment effect on the treated (ATT) (Sant’Anna and Zhao, 2020; Roth et al., 2023).
Second, our findings raise the question of what is the delicate balance between strengthening national science and international collaboration. Collaboration is one of the most remarkable characteristics of contemporary research and international collaboration often yields achievements with greater visibility and higher impact (Adams, 2012; Witze, 2016). In fact, international co-publication has played an indispensable role in China’s rise in science and technology (Cao et al., 2020). While scientists in emerging economies could utilize big-science infrastructures elsewhere, possessing such facilities provides convenience and timely access, ensures accumulation of knowledge and experience, stimulates the participation of competition for priority, and helps establish national leadership in global interdisciplinary and multi-institutional collaboration. Against the current anti-globalization headwind and intensified global technological competition, many governments, especially those in emerging countries, have taken self-strengthening in science as the strategic underpinning for national development. Our study suggests that government-fully funded big-science infrastructures to promote the development of both national and international science, while their effect on increasing the share of high-impact national scientific publications is more prominent. That is, big-science infrastructures are able to help their scientists catch up with the global scientific frontier.
Third, this study measures the impact of big-science infrastructures through the lens of scientific publications. Constrained by data, we could not examine other potential byproducts, such as industrial R&D or scientific networks, which are often associated with such facilities (Autio et al., 2004; Langford and Langford, 2000). These spillovers are certainly important concerning national innovation capability and competitiveness. We encourage future research to collect more nuanced data to study the spillovers of big-science infrastructures.
Finally, there is an ultimate question of whether the scale, concentration, or prestige of investment in big science would really drive a superior scientific return on investment. More generally, can the return of research funding, especially that in big-science infrastructures, be measured, and if so, how (Office of Technology Assessment (OTA), 1986; Stephan, 2012)? In addition to contributing to the advancement of the international frontier of science, the achievement of breakthroughs that would win a country a Nobel Prize, and the generation of cutting-edge technologies, big-science infrastructures add a variety of socioeconomic values, such as the creation of networks and human capital, technology transfer to industry, and regional innovation and development.
Our study adds to these discussions with empirical evidence on the scientific impact of SSRF on knowledge production through the efforts of China-based scientists and international collaboration. It is worth noting that SSRF only represents a typical synchrotron radiation facility. Generalizing our findings to other types of big-science infrastructures should be approached with caution. With the availability of data from more infrastructures and the possibility of combining quantitative and qualitative methods, we encourage future studies to pursue a more comprehensive and holistic examination of the effects of big-science infrastructures.
Data availability
The datasets analyzed during the current study are available in an online open repository (https://osf.io/tq72w).
Notes
-
https://www.nsfc.gov.cn/
References
-
Abramovitz M (1986) Catching up, forging ahead, and falling behind. J Econ Hist 46(2):385–406
Google Scholar
-
Acemoglu D, Aghion P, Zilibotti F (2006) Distance to frontier, selection, and economic growth. J Eur Econ Assoc 4(1):37–74
Google Scholar
-
Adams J (2012) The rise of research networks. Nature 490(7420):335–336
Google Scholar
-
Alberts B (2012) The end of ‘small science’? Science 337(6102):1583
Google Scholar
-
Appelbaum R, Cao C, Han X, Parker R, Simon D (2018) Innovation in China: Challenging the Global Science and Technology System. Polity
-
Autio E, Hameri AP, Vuola O (2004) A framework of industrial knowledge spillovers in big-science centers. Res Policy 33(1):107–126
Google Scholar
-
Beck HP, Charitos P (2021) The Economics of Big Science: Essays by Leading Scientists and Policymakers. Springer
-
Berg J (2017) Science, big and small. Science 358(6370):1504
Google Scholar
-
Cao C, Baas J, Wagner CS, Jonkers K (2020) Returning scientists and the emergence of China’s science system. Sci Public Policy 47(2):172–183
Google Scholar
-
Carrazza S, Ferrara A, Salini S (2016) Research infrastructures in the LHC era: a scientometric approach. Technol Forecast Soc Change 112(2016):121–133
Google Scholar
-
Cramer KC, Hallonsten O, Bolliger IK, Griffiths A (2020) Big science and research infrastructures in Europe: history and current trends. In: Cramer KC, Hallonsten O (eds) Big Science and Research Infrastructures in Europe. Edward Elgar, pp 1–26
-
Crease RP (1999) Making Physics: A Biography of Brookhaven National Laboratory, 1946–1972. University of Chicago Press
-
Crow M (2024) China’s big-science bet. Nature 630(8015):S6–S7
Google Scholar
-
ESFRI (2018) Roadmap 2018: Strategy Report on Research Infrastructures. http://roadmap2018.esfri.eu/media/1066/esfri-roadmap-2018.pdf. Accessed on 3 July, 2023
-
Fleming L, Greene H, Li G, Marx M, Yao D (2019) Government-funded research increasingly fuels innovation. Science 364(6446):1139–1141
Google Scholar
-
Florio M, Sirtori E (2016) Social benefits and costs of large scale research infrastructures. Technol Forecast Soc Change 112:65–78
Google Scholar
-
Fortin JM, Currie DJ (2013) Big science vs. little science: how scientific impact scales with funding. PLoS ONE 8(6):e65263
Google Scholar
-
Furman JL, Stern S (2011) Climbing atop the shoulders of giants: the impact of institutions on cumulative research. Am Econ Rev 101(5):1933–1963
Google Scholar
-
Georghiou L (1998) Global cooperation in research. Res Policy 27:611–626
Google Scholar
-
Guston DH (2000) Retiring the social contract for science. Issues Sci Technol 16(4):32–36
-
Hallonsten O (2013) Introducing ‘facilitymetrics’: a first review and analysis of commonly used measures of scientific leadership among synchrotron radiation facilities worldwide. Scientometrics 96:497–513
Google Scholar
-
Hallonsten O (2014) The politics of European collaboration in big science. In: Mayer M, Carpes M, Knoblich R (eds). The Global Politics of Science and Technology, vol 2. Springer, pp 31–46
-
Hallonsten O (2016) Big Science Transformed: Science, Politics and Organization in Europe and the United States. Palgrave Macmillan
-
Hand E (2010) Big science’ spurs collaborative trend: complicated projects mean that science is becoming ever more globalized-and Europe is leading the way. Nature 463(7279):282–283
Google Scholar
-
Heidler R, Hallonsten O (2015) Qualifying the performance evaluation of Big Science beyond productivity, impact and costs. Scientometrics 104(1):295–312
Google Scholar
-
Helmers C, Overman H (2017) My precious! The location and diffusion of scientific research: evidence from the Synchrotron Diamond Light Source. Econ J 127(604):2006–2040
Google Scholar
-
Holt CA, Sullivan SP (2023) Permutation tests for experimental data. Exp Econ 26:775–812
Google Scholar
-
Howell S (2017) Financing innovation: evidence from R&D grants. Am Econ Rev 107(4):1136–1164
Google Scholar
-
Hwang K (2008) International collaboration in multilayered center-periphery in the globalization of science and technology. Sci Technol Hum Val 33(1):101–133
Google Scholar
-
Jang YS, Ko YJ (2019) How latecomers catch up to leaders in high-energy physics as Big Science: transition from national system to international collaboration. Scientometrics 119(1):437–480
Google Scholar
-
Kevles DJ (1997) Big science and big politics in the United States: reflections on the death of the SSC and the life of the Human Genome Project. Hist Stud 27(2):269–97
-
Kim KW (2006) Measuring international research collaboration of peripheral countries: taking the context into consideration. Scientometrics 66(2):231–240
Google Scholar
-
Langford CH, Langford MW (2000) The evolution of rules for access to mega-science research environments viewed from Canadian experience. Res Policy 29(2):169–179
Google Scholar
-
Li D, Azoulay P, Sampat BN (2017) The applied value of public investments in biomedical research. Science 356(6333):78–81
Google Scholar
-
Martin BR, Irvine J (1981) Internal criteria for scientific choice: an evaluation of research in high-energy physics using electron accelerators. Minerva 19(3):408–32
Google Scholar
-
Martin BR, Irvine J (1983) Assessing basic research: some partial indicators of scientific progress in radio astronomy. Res Policy 12(2):61–90
Google Scholar
-
Nelson RR, Phelps ES (1966) Investment in humans, technological diffusion, and economic growth. Am Econ Rev 56(1/2):69–75
-
OECD (2019) Reference Framework for Assessing the Scientific and socio-economic Impact of Research Infrastructures. https://www.oecd-ilibrary.org/science-and-technology/reference-framework-for-assessing-the-scientific-and-socio-economic-impact-of-research-infrastructures_3ffee43b-en. Accessed on 3 July, 2023
-
Office of Technology Assessment (OTA) (1986) Research Funding as an Investment: Can We Measure the Returns? A Technical Memorandum. U.S. Government Printing Office. https://www.princeton.edu/~ota/disk2/1986/8622/8622.PDF. Accessed 28 Mar 2024
-
Oster E (2019) Unobservable selection and coefficient stability: theory and evidence. J Bus Econ Stat 37(2):187–204
Google Scholar
-
Price DJ (1963) Little Science, Big Science. Columbia University Press
-
Price DJ (1986) Little Science, Big Science … and Beyond. Columbia University Press
-
Roth J, Sant’Anna PH, Bilinski A, Poe J (2023) What’s trending in difference-in-differences? A synthesis of the recent econometrics literature. J Econ 235(2):2218–2244
Google Scholar
-
Smith CL, Borysiewicz L, Casselton L, Conway G, Hassan M (2011) Knowledge, Networks and Nations: Global Scientific Collaboration in the 21st Century. The Royal Society, London
-
Sant’Anna PH, Zhao J (2020) Doubly robust difference-in-differences estimators. J Econ 219(1):101–122
Google Scholar
-
Stephan PE (2012) How Economics Shapes Science. Harvard University Press
-
Traweek S (1992) Beamtimes and Lifetimes: The World of High Energy Physicists. Harvard University Press
-
Wei SX, Wang HY, Cao C, Ye FY (2021) One big science project or 1000 smaller ones? Curr Sci 121(4):479–484
Google Scholar
-
Weinberg AM (1961) Impact of large-scale science on the United States. Science 134(3473):161–164
Google Scholar
-
Witze A (2016) Research gets increasingly international. Nature. https://doi.org/10.1038/nature.2016.19198. Accessed 28 Mar 2024
-
Yang H, Liu L, Wang G (2024) Does large-scale research infrastructure affect regional knowledge innovation, and how? A case study of the National Supercomputing Center in China. Humanit Soc Sci Commun 11(1):1–20
Google Scholar
-
Yang X, Zhou X, Cao C (2023) Remaking the Chinese Academy of Sciences. Science 379(6629):240–243
Google Scholar
-
Zhang J, Vogeley MS, Chen C (2011) Scientometrics of big science: a case study of research in the Sloan Digital Sky Survey. Scientometrics 86(1):1–14
Google Scholar
Acknowledgements
This research is supported by the National Natural Science Foundation of China (71774091 and 72102145), and China’s National Office of Philosophy and Social Sciences (23&ZD149). We thank Douglas Almond, Yi Chen, Yvonne Jie Chen, Hanming Fang, Honghong Li, Xiaoou Liu, Ronald Rousseau, Wei Si, and Richard P. Suttmeier for their comments. We also thank Yang Lu and Haolin Shao for their excellent research assistance.
Author information
Authors and Affiliations
Contributions
XY contributed to study design, data collection and analyses, and manuscript writing. XZ contributed to the literature review and manuscript writing. CC contributed to the study design and manuscript writing.
Corresponding authors
Ethics declarations
Competing interests
The authors declare no competing interests.
Ethical approval
This article does not contain any studies with human participants or their data.
Informed consent
This article does not contain any studies with human participants or their data.
Additional information
Publisher’s note Springer Nature remains neutral with regard to jurisdictional claims in published maps and institutional affiliations.
Supplementary information
Supplementary information
Rights and permissions
Open Access This article is licensed under a Creative Commons Attribution-NonCommercial-NoDerivatives 4.0 International License, which permits any non-commercial use, sharing, distribution and reproduction in any medium or format, as long as you give appropriate credit to the original author(s) and the source, provide a link to the Creative Commons licence, and indicate if you modified the licensed material. You do not have permission under this licence to share adapted material derived from this article or parts of it. The images or other third party material in this article are included in the article’s Creative Commons licence, unless indicated otherwise in a credit line to the material. If material is not included in the article’s Creative Commons licence and your intended use is not permitted by statutory regulation or exceeds the permitted use, you will need to obtain permission directly from the copyright holder. To view a copy of this licence, visit http://creativecommons.org/licenses/by-nc-nd/4.0/.
Reprints and permissions
About this article
Cite this article
Yang, X., Zhou, X. & Cao, C. Beamtimes and knowledge production times: how big-science research infrastructures shape nations’ domestic and international science production.
Humanit Soc Sci Commun 11, 1462 (2024). https://doi.org/10.1057/s41599-024-03993-4
-
Received: 02 April 2024
-
Accepted: 24 October 2024
-
Published: 03 November 2024
-
DOI: https://doi.org/10.1057/s41599-024-03993-4