The Biodiversity Survey of the Cape (BioSCape) in South Africa’s Greater Cape Floristic Region aims to test the limits and potential of remote sensing for biodiversity applications. Integrating field biodiversity measurements and local knowledge with advanced remote sensing, BioSCape brings us closer to measuring key biodiversity variables globally from space.
Addressing biodiversity loss, which jeopardizes human wellbeing and sustainable development, is the target of global conservation and human development goals1. Tracking progress towards these goals requires biodiversity data at a scale and latency that are not feasible for traditional field observations alone. Remote sensing can augment field data and emerging developments, including satellite-borne radar, light detection and ranging (LiDAR) and imaging spectroscopy, have enhanced what can be observed from above. However, because ecosystem properties can be highly site-specific, many biodiversity variables of interest cannot be captured with remote-sensing data exclusively2. To observe the idiosyncrasies of an ecosystem at the spatial and temporal scales necessary to monitor and manage biodiversity, field and remote-sensing datasets must be integrated3.
Advancing the capability of these integrated datasets to measure, monitor and explain patterns and drivers of biodiversity across diverse ecosystems is an urgent priority in the face of the global biodiversity crisis. NASA’s first-ever field campaign focusing on terrestrial, freshwater and marine biodiversity — the Biodiversity Survey of the Cape (BioSCape) — combines local knowledge with global remote-sensing technology to produce openly accessible data products. In this Comment, we introduce BioSCape and emphasize the importance of integrated data and inclusive international collaboration for biodiversity conservation globally.
BioSCape
BioSCape is an integrated remote-sensing and field campaign that was conducted in the Greater Cape Floristic Region (GCFR) of South Africa in October and November of 2023. The GCFR spans eight terrestrial and six marine biomes, hosts two of the world’s richest biodiversity hotspots, and has exceptional levels of terrestrial and aquatic endemism4. Biodiversity in the GCFR is threatened by factors including drought, invasive plants, changes to ocean chemistry and currents, and complex pressures from human populations5,6,7. The GCFR is a microcosm of global challenges — a highly biodiverse region that must support sustainable human development while surviving the threat of climate change. Fortunately, the region has a long history of data-informed conservation and provides learning opportunities that can inform management of similar regions worldwide8.
Integrated data to measure biodiversity
During BioSCape, airborne imaging spectroscopy (also known as hyperspectral remote sensing) and LiDAR data were acquired over terrestrial, freshwater and marine study areas. Imaging spectroscopy measures reflected and emitted solar radiation at high spectral resolution, which enables the retrieval of biodiversity information such as mapping aquatic primary producers or leaf functional traits9,10. In combination with LiDAR measurements of the three-dimensional structure of terrestrial ecosystems, imaging spectroscopy data provide further opportunities for remote sensing of biodiversity, such as classifying tree species11. BioSCape’s airborne dataset, acquired using three imaging spectrometers and two LiDAR instruments, is unprecedented, covering much of the ultraviolet (UV), visible-to-shortwave-infrared (VSWIR) and thermal-infrared (TIR) ranges of the electromagnetic spectrum as well as structural measurements. This combined spectral and structural airborne dataset is necessary to explore the potential of satellite remote sensing for measuring and monitoring global biodiversity12.
BioSCape’s airborne dataset is accompanied by field measurements on land and in water. Field data were collected by 19 individually funded principal-investigator-led research projects, each with its own objectives that included: mapping marine and freshwater biodiversity, including phytoplankton, kelp and harmful algal blooms; quantifying how changes in ocean chemistry and sea-level rise associated with climate change will affect aquatic ecosystems (specifically, estuaries and kelp forests); mapping terrestrial ecosystem properties, including evapotranspiration, plant traits, vegetation 3D structure and mineralogy; quantifying spatial variation and drivers of plant community assembly and ecosystem function, including alien plant invasions; and combining novel field methods, including environmental DNA and acoustic indices, with remotely sensed data to estimate the composition and diversity of biotic communities.
BioSCape’s research projects all aim to better understand the structure, function and composition of the GCFR’s ecosystems, and to learn how and why they are changing in time and space. These diverse projects will integrate and analyse remote-sensing and field data, and advance models and algorithms for measuring and monitoring biodiversity at multiple scales. By combining satellite, airborne and field-based measurements, BioSCape aims to expand the capacity of integrated datasets to produce accurate and scalable findings and help to improve conservation efficacy. High species richness and endemism in the GCFR, combined with open and often scrubby vegetation, make remote sensing particularly challenging. BioSCape will be testing the limits and potential of remote sensing for biodiversity applications worldwide. As a team member noted “if we can do it in the GCFR, we can do it anywhere.”
Inclusive collaboration for increased impact
Applying global perspectives and technology in local contexts requires international collaboration, which can bring benefits including increased scientific impact and applicability13. However, international collaboration can be challenging as countries can have different skills and access to resources. In such cases, extra care must be taken to ensure the collaboration is inclusive and benefits everyone involved.
Since its conception, BioSCape has emphasized creating and maintaining deep and meaningful collaboration between US and South African researchers, and the importance of codeveloping research. The early inclusion of South African researchers in the design of airborne and field components of BioSCape led to a diverse team of about 160 members, of whom approximately half are affiliated with South African institutions and half with US institutions. US participation ensured global applicability and access to best-in-class technology, and bridged gaps in capacity. South African expertise ensured that the research agenda for BioSCape was locally relevant and that local ecological expertise was incorporated.
As biodiversity is intrinsically site-specific, effective conservation hinges on local knowledge and region-specific actions. In the GCFR, strong personal and institutional relationships among research institutes, environmental management authorities and conservation managers have yielded rapid dissemination and uptake of novel science relevant to decision-making. South Africa is a well-known early adopter of systematic conservation planning, has been relatively successful in operationalizing conservation planning into government policy and decision-making, and has ‘set the standard’ for using scientific information in conservation planning and implementation8,14. Therefore, data products created by BioSCape will inform biodiversity conservation, environmental decision-making and policy in South Africa, and guide the management of similar ecosystems worldwide4 (Fig. 1).
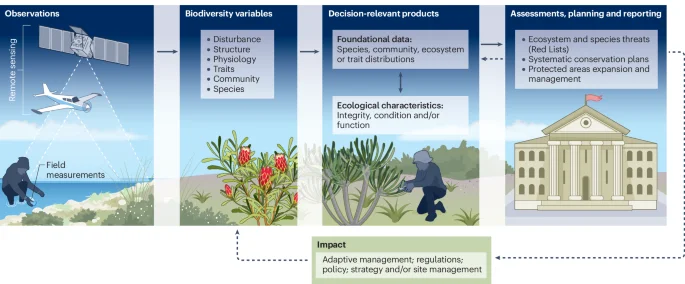
Measuring and monitoring biodiversity variables in campaigns such as BioSCape can directly affect biodiversity conservation. For example, in South Africa, biodiversity observations can be processed to produce relevant data products that are used to inform biodiversity assessment, planning and reporting, which directly affects biodiversity policy and conservation management decisions (which, in turn, inform observation approaches and product development). For this reason, the most impactful biodiversity research projects are codesigned with local stakeholders.
Conclusion
The GCFR’s megadiversity, established pathway from science to application, and diverse threats to biodiversity are a microcosm of global biodiversity challenges and opportunities. As satellite acquisitions of rich, highly dimensional data types improve in area coverage, spatial resolution and temporal latency in the coming years, BioSCape provides a proof of concept for remote sensing of biodiversity using imaging spectroscopy and LiDAR combined with several complementary field techniques. Although some of BioSCape’s planned approaches might fail, we expect they will teach us almost as much about the remote sensing of biodiversity as the successes — not to mention the serendipitous discoveries.
References
-
Conference of the Parties to the Convention on Biological Diversity. 15/5. Monitoring Framework for the Kunming-Montreal Global Biodiversity Framework (2022).
-
Cavender-Bares, J. et al. Integrating remote sensing with ecology and evolution to advance biodiversity conservation. Nat. Ecol. Evol. 6, 506–519 (2022).
Google Scholar
-
Turner, W. Sensing biodiversity. Science 346, 301–302 (2014).
Google Scholar
-
Skowno, A. et al. National Biodiversity Assessment 2018: The Status of South Africa’s Ecosystems and Biodiversity. Synthesis Report (South African National Biodiversity Institute, an entity of the Department of Environment, Forestry and Fisheries, 2019).
-
James, N. et al. Effects of climate change on South African estuaries and associated fish species. Clim. Res. 57, 233–248 (2013).
Google Scholar
-
Slingsby, J. A. et al. Intensifying postfire weather and biological invasion drive species loss in a Mediterranean-type biodiversity hotspot. Proc. Natl Acad. Sci. USA 114, 4697–4702 (2017).
Google Scholar
-
Sydeman, W. J. et al. Climate change and wind intensification in coastal upwelling ecosystems. Science 345, 77–80 (2014).
Google Scholar
-
Balmford, A. Conservation planning in the real world: South Africa shows the way. Trends Ecol. Evol. 18, 435–438 (2003).
Google Scholar
-
Hestir, E. & Dronova, I. Remote sensing of primary producers in the Bay–Delta. SFEWS 20, article 5 (2023).
-
Wang, Z. et al. Foliar functional traits from imaging spectroscopy across biomes in eastern North America. New Phytol. 228, 494–511 (2020).
Google Scholar
-
Naidoo, L., Cho, M. A., Mathieu, R. & Asner, G. Classification of savanna tree species, in the Greater Kruger National Park region, by integrating hyperspectral and LiDAR data in a random forest data mining environment. ISPRS J. Photogramm. Remote Sens. 69, 167–179 (2012).
Google Scholar
-
Dierssen, H. M. et al. Synergies between NASA’s hyperspectral aquatic missions PACE, GLIMR, and SBG: opportunities for new science and applications. JGR Biogeosci. 128, e2023JG007574 (2023).
Google Scholar
-
Choi, H. & Oh, D. The importance of research teams with diverse backgrounds: research collaboration in the Journal of Productivity Analysis. J. Prod. Anal. 53, 5–19 (2020).
Google Scholar
-
Buschke, F. T., Botts, E. A. & Sinclair, S. P. Post‐normal conservation science fills the space between research, policy, and implementation. Conserv. Sci. Pract. 1, e73 (2019).
Google Scholar
Acknowledgements
The authors acknowledge the invaluable contributions of the entire BioSCape team, including scientists, engineers, field technicians, data analysts and support staff. The US National Aeronautics and Space Administration (NASA) funded the US components of the BioSCape campaign including airborne remote-sensing instruments through NASA grant no. 80NSSC21K0086 to A.M.W. and E.L.H. J.A.S. and G.R.M. were funded through National Research Foundation grant no. 142438 and the South African Environmental Observation Network (SAEON). We further acknowledge the contributions of the South African National Research Foundation (NRF), which funded two affiliated South African-led research projects. The SAEON provided in-kind support, access to research platforms and sites, and supported several capacity-building activities. J.A.S. further thanks UNESCO and the Government of The Netherlands for providing additional funding for capacity building and support for young South African researchers. The BioSCape project was also supported by NASA projects no. NNX16AQ45G to A.M.W., no. NNX16AQ34G to E.L.H., and no. 21-SMDSS21-0041 to P.G.B. J.N. was supported by the National Science Foundation Graduate Research Fellowship Program (NSF-GRFP) no. 2139297.
Author information
Authors and Affiliations
Consortia
Contributions
A.W.C. contributed to conceptualization; methodology; investigation; writing (original draft); writing (review and editing); visualization; project administration; and funding acquisition. E.L.H. contributed to conceptualization; methodology; investigation; writing (reviewing and editing); visualization; resources; supervision; project administration; and funding acquisition. J.A.S. contributed to conceptualization; methodology; investigation; writing (review and editing); and project administration. C.J.F. contributed to writing (review and editing). G.R.M. contributed to conceptualization; investigation; and writing (review and editing). A.L.S. contributed to writing (review and editing); and visualization. W.T. contributed to writing (review and editing); conceptualization; methodology; resources; supervision; project administration; and funding acquisition. K.D.G. contributed to conceptualization; methodology; resources; supervision; project administration; and funding acquisition. P.G.B. contributed to methodology; investigation; and writing (reviewing and editing). J.N. contributed to investigation; writing (review and editing); and visualization. A.M.W. contributed to conceptualization; methodology; investigation; resources; writing (review and editing); visualization; supervision; project administration; and funding acquisition.
Corresponding author
Ethics declarations
Competing interests
The authors declare no competing interests.
Supplementary information
Supplementary information
Rights and permissions
Reprints and permissions
About this article
Cite this article
Cardoso, A.W., The BioSCape Consortium. BioSCape combines local knowledge and remote-sensing technology for inclusive biodiversity science.
Nat. Rev. Biodivers. 1, 2–4 (2025). https://doi.org/10.1038/s44358-024-00007-8
-
Published: 15 January 2025
-
Issue Date: January 2025
-
DOI: https://doi.org/10.1038/s44358-024-00007-8