Abstract
Large-scale tsunamis destroy coastal areas and rapidly transport huge amounts of plants and other debris over long distances. However, due to their poor preservation potential and the lack of unequivocal identifying features, tsunami deposits are rarely recognized in the geological record except for geologically young Holocene coastal deposits. This study focuses on pelagic settings as potential archives of large-scale paleo-tsunami events. Here we describe extraordinarily rich amber concentrations in Early Cretaceous deep sea deposits (116–114 Ma). The amber is distinctively deformed in a manner comparable to typical soft-sediment deformation structures such as flame structures. As resin exposed to the air hardens quickly in weeks, the flame deformation of the resin suggests that it reached pelagic seafloors without significant subaerial exposure. This new observation of amber as a soft-sediment unveils the whole sedimentary process from erosion to burial, a view neglected by previous sedimentological studies that focused on clastic and carbonate sediments. The most plausible cause for the presence of this enigmatic amber in a deep-sea setting is large-scale tsunamis, which are supported by the mode of occurrence of amber, associated sedimentary structures, and a massive coinstantaneous landslide.
Similar content being viewed by others
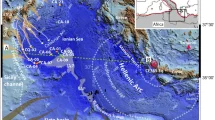
Recognizing megatsunamis in Mediterranean deep sea sediments based on the massive deposits of the 365 CE Crete event
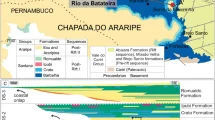
Early Aptian marine incursions in the interior of northeastern Brazil following the Gondwana breakup
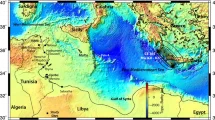
Tsunami deposits in Tunisia contemporaneous of the large 365 CE Crete earthquake and Mediterranean Sea catastrophic event
Introduction
Tsunamis are catastrophic sea waves mainly caused by dramatic submarine/coastal crustal shifts and asteroid impacts1. They attract attention from the standpoint of disaster prevention and have therefore been studied intensively in Holocene coastal deposits2. With the exception of asteroid deposits3,4, the unambiguous recognition of pre-Holocene paleo tsunamis is very difficult for two significant reasons. Firstly, coastal tsunami deposits are easily eroded in that dynamic environment1, and secondly, robust criteria for tsunami deposits are unestablished due to the difficulty of distinguishing tsunamis from other high-energy coastal events (e.g. cyclones; see5).
Here we report potential large-scale paleo-tsunami deposits in the Early Cretaceous deep pelagic sediments in northern Japan (116–114 Ma). We describe extraordinarily rich amber (fossilized resin) concentrations in a siliciclastic succession. We focus on soft-sediment deformations such as flame structures formed by resin. Fresh resin hardens rapidly in the air but keeps malleable underwater6. Resin deposits with soft-sediment deformation show rapid transportation from land to sea floors without air-exposed conditions. The novel perspective of resin as a soft-sediment constrains its transportation process, which cannot be recorded directly from clastic/carbonate sediments, and reveals the large-scale paleo-tsunamis archived in deep pelagic sea settings.
Geological setting
The geology of Hokkaido Island, northern Japan, is characterized by Cretaceous–Paleogene forearc basin deposits (Yezo Group) which are resting on the oceanic crust (Sorachi Group) along the eastern margin of the Eurasian continent (Fig. 1;7,8,9). The Sorachi Group (total thickness ca. 1600 m) is divided into a basaltic lower part and a volcaniclastic upper part. The overlying Yezo Group is composed of a thick terrestrially influenced, > 10 km siliciclastic succession10. The lower part of the Yezo Group, which conformably overlies volcanogenic and siliceous deposits (e.g. chert and felsic tuff) of the upper Sorachi Group, was considered to be formed in deep sea settings10. Macro-fossils with carbonate shells such as ammonites are extremely rare in the lower part of the Yezo Group.
The lithostratigraphic change between the Sorachi and Yezo groups is interpreted as a consequence of tectonic activities through the latest Jurassic to Early Cretaceous at the eastern margin of the Eurasian continent11. The westward dipping subduction of the Izanagi-Kula plate caused the uplift of the hinterland, the formation of the large forearc basin, and onset of the terrigenous sediment supply system11,12. Enigmatic amber-rich siliciclastic deposits occur directly above the boundary of the Sorachi and Yezo groups in the Shimonakagawa Quarry (44.827722, 142.112528), Nakagawa area, northern Hokkaido (Fig. 1).
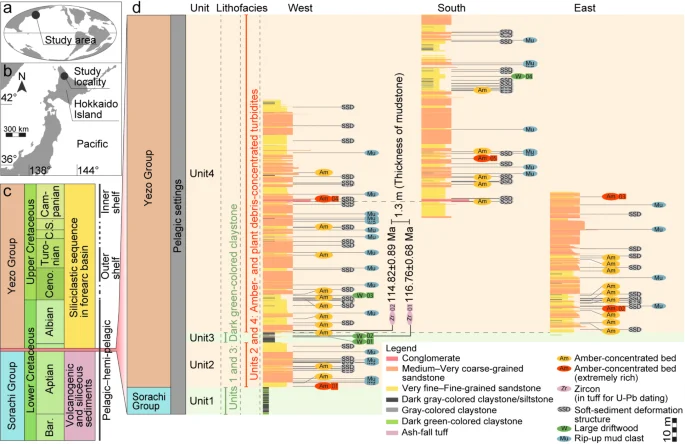
Locality and stratigraphic distribution of amber-concentrated beds in the Shimonakagawa Quarry, Nakagawa area, Hokkaido, northern Japan. a, b Locality map. Early Cretaceous paleomap is modified from9. c Stratigraphic scheme and a general trend of depositional settings of the Cretaceous siliciclastic sequence in the Nakagawa area, modified from9. Bar.–Barremian. Ceno.–Cenomanian. C.–Coniacian. S.–Santonian. d Columnar sections in the west, south, and east portions of the quarry (Supplementary Fig. S1). The horizons of amber high-concentrated beds, tuff layers used for zircon U-Pb dating and large driftwood are shown with each bed number.
Materials and methods
U-Pb age dating
The background sedimentation rate of the depositional setting was reconstructed by U-Pb dating. Zircon grains were collected from two thin ash-fall tuff layers (Zr 01 and Zr 02 in Fig. 1) and analyzed by sensitive high-resolution ion microprobe (SHRIMP II-e; Korean Basic Science Institute). The background sedimentation rate was calculated by differences in U-Pb ages of the two tuff layers and the thickness of claystone between them (1.3 m), excluding turbidites. Details of SHRIMP procedures are provided in Supplementary Note S1.
Sedimentological field survey and visualization of internal structures of amber
In the field, stratigraphic distribution, amber-bearing beds, and associated sedimentary structures were described in detail. Internal deformation structures of the amber were visualized by a novel imaging technique, fluorescence grinding tomography (Paleobiology lab, Hokkaido University). Rock samples of amber-concentrated beds of Am 01 and Am 02 were ground down by an automatic grinder and the exposed surface was photographed with a high-resolution digital camera (50.6-megapixel) in 40 μm intervals (the total thickness of 20.12 mm and 25.12 mm, respectively). Each photo was taken under white and UV (wavelength 365 nm) lights because the strong autofluorescence of amber is very useful for visualizing its structures (Fig. 2e). Detailed procedures and all data sequentially arranged are shown in Supplementary Note S2, Supplementary Table S1, and Supplementary Videos S1–S3.
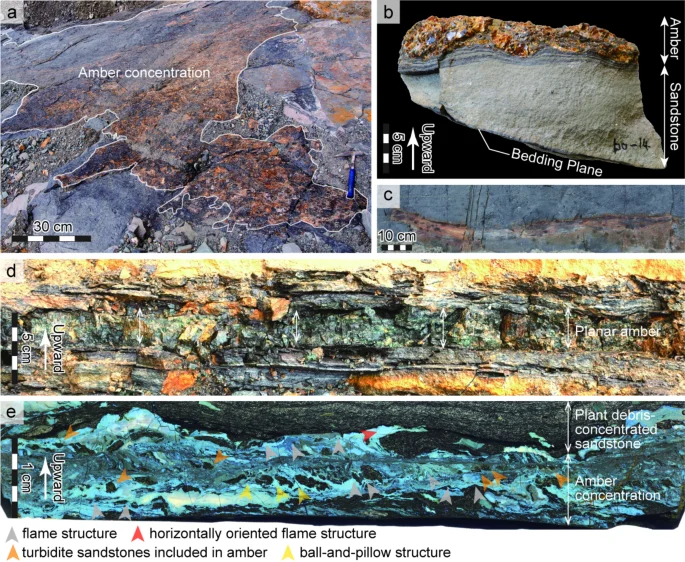
The mode of occurrences of amber and associated sedimentary structures. a, b Amber concentration in Am 02 widely exposed on the bedding plane. c A large driftwood in claystone bed of W 02. d, e A planar-shaped amber in Am 01. e A fluorescence tomogram (NMNSDS00047_c1_wluv365_01_0003) showing soft-resin deformation structures. Cross section. Photographed simultaneously under UV and white lights. Stratigraphically upward is the proximal side of the surfaces in photographs (a–c). See Fig. 1d for horizons of a–e.
Results
Lithofacies
In the Shimonakagawa Quarry, Nakagawa area, northern Hokkaido, the approximately 150 m thick sequence is divided into four lithological units (Fig. 1d; Supplementary Fig. S1). Units 1 and 3 are condensed, characterized by gray- and dark-green, unbioturbated claystones with ash-fall tuffs and well-developed parallel laminations of radiolarians. Units 2 and 4 are composed of alternating beds of sandstone and dark gray-colored siltstone. Amber and plant debris are concentrated in the sandstone beds (Fig. 2; Supplementary Fig. S2). Unit 1 and Units 2–4 are correlated to the Sorachi and Yezo Groups, respectively.
Units 2 and 4 of the sequence consist of alternating beds of sandstone and dark gray-colored siltstone. More than 400 sandstone beds are identified and are mainly divided into two lithofacies. Type 1 sandstones typically show normal grading from medium- to very fine-grained, interpreted as distal turbidites. Type 2 sandstones are poorly sorted, silty to fine-grained, interpreted as cohesive debris-flow deposits13. Type 1 sandstones, which make up most sandstone beds in Units 2 and 4, are about 0.5 cm–6.8 m thick (Supplementary Fig. S1). Their thickness changes laterally, and some beds thin out over a distance of 100 m. Thinning upward cycles occur every 5–20 m. Each sandstone bed typically exhibits into three vertical divisions. The bottom division (0–160 cm) is generally flat at its base without an erosional surface. The middle division (0–630 cm) is massive and rarely shows grading. The upper division (0–95 cm) is graded upward and generally contains parallel lamination of plant debris. In the bottom to medium divisions, subangular to subrounded, coarse- to very coarse-grained clasts are occasionally observed. Pebble-sized clasts (7 mm in maximum diameter) are rare. Type 2 sandstones are rarely intercalated in the sequence and are about 7–90 cm thick (Supplementary Fig. S1). Unlaminated plant debris and rip-up mud clasts are concentrated.
Background sedimentation rate
The weighted mean of the 206Pb/ 238U zircon ages of two tuff layers (Zr 01, Zr 02) are 116.76 ± 0.68 Ma (n = 33, MSWD = 1.8) and 114.82 ± 0.89 Ma (n = 61, mean square weighted deviation; MSWD = 2.5), respectively (Supplementary Note S4, Supplementary Figs. S3 and S4, and Supplementary Table S2). These data reveal a latest Aptian age14 for these sediments and allow us to calculate the background sedimentation rate of the sequence, which is 0.38–3.66 mm/kyr.
Stratigraphic distribution and the mode of occurrences of amber
Thirty amber-bearing beds, with thicknesses of 2–270 cm, were recognized in the sandstone beds of Units 2 and 4 (Fig. 1). In five of these (Am 01 to 05), amber occupied as much as 80% of exposed surfaces in cross-section or on bedding planes (Figs. 1 and 2). Amber is especially abundant in Am 01 (3 cm-thick of amber on average) and Am 02 (3–7 cm in thickness). Am 02 is widely exposed on a 10 m × 10 m sized bedding plane (Fig. 2a). Am 01 is the lowest bed of the Yezo Group (Fig. 1).
Resin deformation in amber
Amber is mostly concentrated in the upper division of the sandstone beds of Units 2 and 4 (Fig. 1d). The shapes of amber are mainly classified into lump and planar forms. The lump forms of amber are shown as spherical pieces (< 1 cm to 3 cm in diameter) and angular to sub-angular fragments (< 1 mm to 5 mm in diameter), and commonly occur with plant debris. The planar forms of amber are horizontally continuous and complexly deformed (Fig. 2d and e and Supplementary Figs. S5 and S6). The planar amber is mixed with mud and turbidite sand sediments (Fig. 2e and Supplementary Fig. S6). It also fills up the surrounding spaces of other amber fragments and pieces (Supplementary Fig. S6). The planar amber shows distinctive internal structures similar to typical soft-sediment deformation structures such as flame structures (Figs. 2e and Supplementary Fig. S5). At the interface between the amber and siliciclastic sediments in Am 01, upward-projecting flame and ball-and-pillow structures are well-developed. Some projections extend horizontally.
Amber includes protist-like forms, pollen grains, acarids and insects (Supplementary Fig. S7). The protist-like forms, which are here interpreted as phloem sap15, are deformed along the direction of resin flow. The pollen and exoskeletal inclusions are undeformed.
Sedimentary structures associated with amber-concentrated beds
Various sedimentary structures (Supplementary Figs. S8–S10), most of which have been proposed as tsunami deposits by previous studies1, are commonly described in Units 2–4.
Abundant plant debris (Fig. 2e and Supplementary Fig. S8): The upper division of sandstone beds in Units 2 and 4 contain abundant plant debris, ranging in length from less than 1 mm to 30 mm.
Large driftwood (Fig. 2c; W in Fig. 1d): Robust wood fossils (over 30 cm and up to 1.3 m long) occur sporadically in sandstone beds and claystone. They show clearly different sizes compared to the plant debris in the same layer. They are found isolated in the claystone layers, not only in the plant-concentrated layers. They lack bio-erosion by shallow-marine benthic boring organisms.
Large rip-up clasts (Mu in Fig. 1d): Large rip-up mud clasts (over 1 m in maximum diameter) frequently occur in sandstone beds in Units 2 and 4. Mud clasts are very angular to well-rounded and typically consist of dark gray-colored siltstone.
Soft-sediment deformation structures (SSD in Fig. 1d): Various soft-sediment deformation structures (millimeter to meter-scale), such as sand injections, small-scale diapirs, flame structures, complex convolutions, slump structures, and tightly folding structures, are common in Units 2 and 4.
Discussion and conclusion
Most sandstones in Units 2 and 4 are interpreted as distal turbidites. Well-developed laminations of radiolarian skeletons and ash-fall tuffs in the claystone of Units 1 and 3 suggest low-energy sedimentation. The background sedimentation rates proposed here (0.38–3.66 mm/kyr) are concordant with those of pelagic bedded cherts (0.4–5.3 mm/kyr) estimated in previous studies (Supplementary Note S3 and Supplementary Table S3). All of these suggest that the extraordinarily rich amber accumulated in a pelagic environment.
Though over one hundred amber localities have been reported from the Upper Carboniferous–the Upper Pleistocene, they are restricted to terrestrial and shallow-marine settings16,17. Abundant amber in the pelagic settings reported here implies the existence of exceptional sedimentary processes.
Amber generally forms drop, tube, or spherical shapes18 because of the unique nature of resin that rapidly hardens by the loss of volatiles subaerially16. Schmidt & Dilcher7 showed that modern resin hardens in about one week after exudation; in contrast, the resin flowing into water keeps soft unless exposed to the air.
In our study, horizontally continuous planar amber has distinctive internal structures similar to flame and ball-and-pillow structures (Fig. 2e). Such structures are generally observed in siliciclastics and formed by “soft-sediment” deformation19. The formation of these structures in amber strongly suggests that the resin was still soft when deposited. Vertically projecting flame and ball-and-pillow structures of the amber are considered to be formed by density-inversion at the interface of resin and sediments. Horizontally-oriented amber projections, which are similar to truncated flame structures20, indicate that, at deposition, the resin could still be deformed by water flow. The horizontally continuous planar amber (Fig. 2) supports that soft-resin flowed and spread viscously into the seafloor. Late diagenetic deformation of amber is excluded by the following observations: (1) deformed planar amber and angular to subangular amber fragments in the same layers (Supplementary Fig. S6), and (2) co-occurrence of deformed liquid inclusions (e.g., protist-like forms) and undeformed hard bio-inclusions (e.g., arthropods). These resin deformations occurred underwater, implying their direct transport from the forest to the pelagic seafloor. Such rapid and direct transport of terrestrial materials from land to ocean could be driven by a tsunami.
The following indicators observed in the studied sequence point to tsunami-related deposition1: (a) large amounts of plant debris are supplied from land forest destruction; (b) mega mud clasts, apparently ripped up by destruction and erosion of seafloor; (c) soft-sediment liquefaction and slump deformation; and (d) Large driftwood trunks lacking bio-erosion by shallow-marine benthic boring organisms, indicating rapid transportation without time to stack. The occurrence of the trunks in the claystone (Fig. 2c) indicates that the enormous number of wood was discharged, drifted, and sank to the pelagic sea floor, not transported by turbidity currents.
Other possible events which supply terrigenous material into the ocean are storms and floods. Storm waves, however, would not affect pelagic bottoms. Although the flood-induced hyperpycnal flows can transport plant remains into marine settings, sedimentary structures such as typical of such processes, including coarsening upward sequences with erosional surfaces21, are not observed here. These lines of evidence suggest tsunamis as a possible cause of a large amount of soft resin transportation into the pelagic setting.
The boundary between the Sorachi and Yezo groups is marked by the formation of a large forearc basin and hinterland uplifts with the subduction of the Izanagi-Kula plates12). This change is significant throughout the Cretaceous of northern Japan. Because a total of 30 amber-bearing beds are intercalated in the lowermost part of the Yezo Group (Units 2 and 4), deposition of the Yezo Group thus began with the massive supply of amber (Am 01 in Figs. 1d and 2d and e), an occurrence that may be tied to tectonic instability.
A catastrophic landslide deposit containing huge sheet-like blocks of shallow-marine reef carbonates (maximum 60 m thick and at least 3 km long) is documented from the lower part of the Yezo Group in central Hokkaido, 200 km to the south of the study area. Previous studies8,10,22 have proposed that a shallow-marine carbonate platform, the largest throughout the Jurassic–Cretaceous of Northwest Pacific, collapsed and its blocks were transported into a deeper part of the basin in the latest Aptian. These events were correlated with debris-flow deposits, including abundant limestone pebbles in the basal Yezo Group in the Nakagawa area by larger benthic foraminiferal biostratigraphy8,22. The debris-flow deposits occur 10 km to the south of the Shimonakagawa Quarry and can be correlated with Unit 2. This suggests two devastating, concurrent events; (1) forest destruction and mass transport of amber into the deep sea, and (2) shallow-marine carbonate platform collapse. Therefore, it is plausible that major earthquakes accompanied by tsunamis occurred in the latest Aptian of the eastern margin of Eurasia.
In summary, we reconstruct several phases of the latest Aptian tectonism in the northeast Pacific (Fig. 3): (1) the occurrence of major earthquakes and tsunamis; (2) the complete destruction of forested coastal settings, (3) the discharge of a large amount of plant debris, fresh resin, and siliciclastic sediments into the ocean, (4) direct delivery to the deep sea floor by tsunami-induced turbidity currents; while simultaneously, (5) wood and resin drifted on the sea surface, which (6) later sank to seafloors; and finally (7) repetition of such tsunami events. We propose that large-scale tsunamis may not be recorded in coastal areas due to the destruction of such settings, but pelagic sediments can serve as significant archives for large-scale destructive events linking forests and pelagic realm.
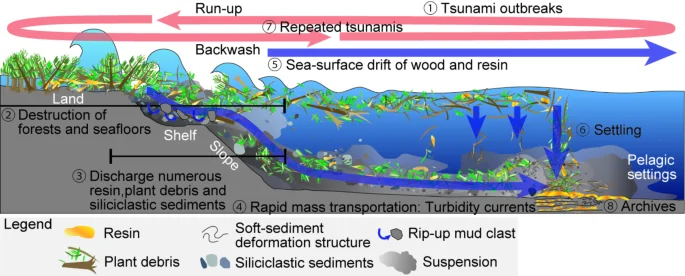
Reconstruction of Early Cretaceous large-scale tsunamis which reached pelagic deep-sea bottoms from lands. (1) Outbreaks of earthquakes and tsunamis. (2) Destruction of extensive forests and seafloors by tsunamis and (3) Discharge of numerous fresh resin, plant debris and siliciclastic sediments into the ocean. (4) Direct and rapid transportation into deep sea bottoms by tsunami-induced turbidity currents. (5) Wood and resin drift and (6) late settling. (7) Repeated tsunamis during tectonic instability, and (8) its archives in pelagic strata.
Data availability
All data are included in the manuscript and supplementary information files.
References
-
Dawson, A. G. & Stewart, I. Tsunami deposits in the geological record. Sediment. Geol. 200, 166–183. https://doi.org/10.1016/j.sedgeo.2007.01.002 (2007).
Google Scholar
-
Costa, P. J. & Andrade, C. Tsunami deposits: present knowledge and future challenges. Sedimentology 67, 1189–1206. https://doi.org/10.1111/sed.12724 (2020).
Google Scholar
-
Bourgeois, J., Hansen, T. A., Wiberg, P. L. & Kauffman, E. G. A tsunami deposit at the Cretaceous-Tertiary boundary in Texas. Science 241, 567–570. https://doi.org/10.1126/science.241.4865.567 (1988).
Google Scholar
-
Paris, R., Goto, K., Goff, J. & Yanagisawa, H. Advances in the study of mega-tsunamis in the geological record. Earth Sci. Rev. 210, 103381. https://doi.org/10.1016/j.earscirev.2020.103381 (2020).
Google Scholar
-
Shanmugam, G. Process-sedimentological challenges in distinguishing paleo-tsunami deposits. Nat. Hazards. 63, 5–30. https://doi.org/10.1007/s11069-011-9766-z (2012).
Google Scholar
-
Schmidt, A. R. & Dilcher, D. L. Aquatic organisms as amber inclusions and examples from a modern swamp forest. Proc. Natl. Acad. Sci. USA 104, 16581–16585. https://doi.org/10.1073/pnas.0707949104 (2007)
-
Ueda, H. Hokkaido in The Geology of Japan (eds Moreno, T., Wallis, S., Kojima, T., & Gibbons, W.) 201–221 (Geological Society, 2016) https://doi.org/10.1144/GOJ.8
-
Iba, Y. & Sano, S. Mid-Cretaceous step-wise demise of the carbonate platform biota in the Northwest Pacific and establishment of the North Pacific biotic province. Palaeogeogr. Palaeoclimatol. Palaeoecol. 245, 462–482. https://doi.org/10.1016/j.palaeo.2006.09.008 (2007).
Google Scholar
-
Iba, Y. et al. Belemnite extinction and the origin of modern cephalopods 35 m.y. prior to the Cretaceous–Paleogene event. Geology 39, 483–486. https://doi.org/10.1130/G31724.1 (2011).
Google Scholar
-
Takashima, R. et al. Geology and stratigraphy of forearc basin sediments in Hokkaido, Japan. Cretaceous environmental events on the north-west Pacific margin. Cretac. Res. 25, 365–390. https://doi.org/10.1016/j.cretres.2004.02.004 (2004).
Google Scholar
-
Niida, K. & Kito, N. Cretaceous arc-trench systems in Hokkaido. Association Geol. Collab. Japan Monogr. 31, 379–402 (1986). (in Japanese with English abstract).
-
Kiminami, K., Komatsu, M. & Kawabata, K. Composition of clastics of the Sorachi Group and the Lower Yezo group in the Inushibetsu-gawa area, Hokkaido, Japan, and their significance. Mem. Geol. Soc. Japan. 38, 1–11 (1992). (in Japanese with English abstract).
-
Naruse, H. Cretaceous to Paleocene depositional history of North-Pacific subduction zone: reconstruction from the Nemuro Group, eastern Hokkaido, northern Japan. Cretac. Res. 24, 55–71. https://doi.org/10.1016/S0195-6671(03)00024-7 (2003).
Google Scholar
-
Gradstein, F. M. & Ogg, J. G. The chronostratigraphic scale. Geol. Time Scale 21–32. https://doi.org/10.1016/B978-0-12-824360-2.00002-4 (2020).
-
Lozano, R. P. et al. Phloem sap in cretaceous ambers as abundant double emulsions preserving organic and inorganic residues. Sci. Rep. 10, 9751. https://doi.org/10.1038/s41598-020-66631-4 (2020).
Google Scholar
-
Langenheim, J. H. Plant Resins: Chemistry, Evolution, Ecology, and Ethnobotany (Timber, 2003).
-
Martı́nez-Delclòs, X., Briggs, D. E. G. & Peñalver, E. Taphonomy of insects in carbonates and amber. Palaeogeogr. Palaeoclimatol. Palaeoecol. 203, 19–64. https://doi.org/10.1016/S0031-0182(03)00643-6 (2004).
Google Scholar
-
Seyfullah, L. et al. Production and preservation of resins–past and present. Biol. Rev. 93, 1684–1714. https://doi.org/10.1111/brv.12414 (2018).
Google Scholar
-
Owen, G. Load structures: gravity-driven sediment mobilization in the shallow subsurface. Geol. Soc. Spec. Publ. 216, 21–34. https://doi.org/10.1144/GSL.SP.2003.216.01.03 (2003).
Google Scholar
-
Matsumoto, D. et al. Truncated flame structures within a deposit of the Indian Ocean Tsunami: evidence of syn-sedimentary deformation. Sedimentology 55, 1559–1570. https://doi.org/10.1111/j.1365-3091.2008.00957.x (2008).
Google Scholar
-
Mulder, T. & Alexander, J. The physical character of subaqueous sedimentary density flows and their deposits. Sedimentology 48, 269–299. https://doi.org/10.1046/j.1365-3091.2001.00360.x (2001).
Google Scholar
-
Iba, Y. & Sano, S. Mesorbitolina (Cretaceous larger foraminifera) from the Yezo Group in Hokkaido, Japan and its stratigraphic and paleobiogeographic significance. Proc. Japan Acad. Ser. B. 82, 216–223. https://doi.org/10.2183/pjab.82.216 (2006).
Google Scholar
Acknowledgements
We thank Y. Hikida, N. Tohyama, Y. Adachi, and M. Murakami for their help in the fieldwork. T. Ishii, T. Tajima, S. Sasaki, S. Ikegami, and T. Ozaki for assisting in visualization of the internal structures of amber. Thanks are extended to D.E. Fastovsky for the critical reading of the manuscript.
Funding
This research was supported by the Japan Society for the Promotion of Science (grant no. 23K27235 for A.K., 23K17274 for Y.T, 19H02010 and 22H02937 for Y.I.), and Japan Aerospace Exploration Agency (grant no. JX-PSPC-540452 for Y.I.).
Author information
Authors and Affiliations
Contributions
Conceptualization: A.K. Methodology: A.K., Y.T., K.Y., S.S., and Y.I. Investigation: A.K. Funding acquisition: A.K., Y.T., and Y.I. Supervision: Y.I. Writing—original draft: A.K. Writing—review and editing: All authors.
Corresponding author
Ethics declarations
Competing interests
The authors declare no competing interests.
Additional information
Publisher’s note
Springer Nature remains neutral with regard to jurisdictional claims in published maps and institutional affiliations.
Electronic supplementary material
Below is the link to the electronic supplementary material.
Supplementary Material 1
Supplementary Material 2
Supplementary Material 3
Supplementary Material 4
Rights and permissions
Open Access This article is licensed under a Creative Commons Attribution-NonCommercial-NoDerivatives 4.0 International License, which permits any non-commercial use, sharing, distribution and reproduction in any medium or format, as long as you give appropriate credit to the original author(s) and the source, provide a link to the Creative Commons licence, and indicate if you modified the licensed material. You do not have permission under this licence to share adapted material derived from this article or parts of it. The images or other third party material in this article are included in the article’s Creative Commons licence, unless indicated otherwise in a credit line to the material. If material is not included in the article’s Creative Commons licence and your intended use is not permitted by statutory regulation or exceeds the permitted use, you will need to obtain permission directly from the copyright holder. To view a copy of this licence, visit http://creativecommons.org/licenses/by-nc-nd/4.0/.
Reprints and permissions
About this article
Cite this article
Kubota, A., Takeda, Y., Yi, K. et al. Amber in the Cretaceous deep sea deposits reveals large-scale tsunamis.
Sci Rep 15, 14298 (2025). https://doi.org/10.1038/s41598-025-96498-2
-
Received: 22 December 2024
-
Accepted: 28 March 2025
-
Published: 15 May 2025
-
DOI: https://doi.org/10.1038/s41598-025-96498-2
Keywords
- Fossil resin
- Soft-sediment deformation structures
- Forearc basin
- Cretaceous
- U-Pb dating
- Fluorescence grinding tomography