Abstract
Providing molecular fingerprint information, vibrational spectroscopic imaging opens a new window to decipher the function of biomolecules in living systems. While classic vibrational microscopes based on spontaneous Raman scattering or mid-infrared absorption offer rich insights into sample composition, they have very small cross sections or poor spatial resolution. Nonlinear vibrational microscopy, based on coherent Raman scattering or optical photothermal detection of vibrational absorption, overcomes these barriers and enables high-speed and high-sensitivity imaging of chemical bonds in live cells and tissues. Here, we introduce various modalities, including their principles, strengths, weaknesses and data mining methods to the life sciences community. We further provide a guide for prospective users and an outlook on future technological advances.
This is a preview of subscription content, access via your institution
Access options
/* style specs end */
Access Nature and 54 other Nature Portfolio journals
Get Nature+, our best-value online-access subscription
$29.99 / 30 days
cancel any time
Subscribe to this journal
Receive 12 print issues and online access
$259.00 per year
only $21.58 per issue
Buy this article
- Purchase on SpringerLink
- Instant access to full article PDF
Prices may be subject to local taxes which are calculated during checkout
/* style specs start */
style {
display: none !important;
}
.LiveAreaSection * {
align-content: stretch;
align-items: stretch;
align-self: auto;
animation-delay: 0s;
animation-direction: normal;
animation-duration: 0s;
animation-fill-mode: none;
animation-iteration-count: 1;
animation-name: none;
animation-play-state: running;
animation-timing-function: ease;
azimuth: center;
backface-visibility: visible;
background-attachment: scroll;
background-blend-mode: normal;
background-clip: borderBox;
background-color: transparent;
background-image: none;
background-origin: paddingBox;
background-position: 0 0;
background-repeat: repeat;
background-size: auto auto;
block-size: auto;
border-block-end-color: currentcolor;
border-block-end-style: none;
border-block-end-width: medium;
border-block-start-color: currentcolor;
border-block-start-style: none;
border-block-start-width: medium;
border-bottom-color: currentcolor;
border-bottom-left-radius: 0;
border-bottom-right-radius: 0;
border-bottom-style: none;
border-bottom-width: medium;
border-collapse: separate;
border-image-outset: 0s;
border-image-repeat: stretch;
border-image-slice: 100%;
border-image-source: none;
border-image-width: 1;
border-inline-end-color: currentcolor;
border-inline-end-style: none;
border-inline-end-width: medium;
border-inline-start-color: currentcolor;
border-inline-start-style: none;
border-inline-start-width: medium;
border-left-color: currentcolor;
border-left-style: none;
border-left-width: medium;
border-right-color: currentcolor;
border-right-style: none;
border-right-width: medium;
border-spacing: 0;
border-top-color: currentcolor;
border-top-left-radius: 0;
border-top-right-radius: 0;
border-top-style: none;
border-top-width: medium;
bottom: auto;
box-decoration-break: slice;
box-shadow: none;
box-sizing: border-box;
break-after: auto;
break-before: auto;
break-inside: auto;
caption-side: top;
caret-color: auto;
clear: none;
clip: auto;
clip-path: none;
color: initial;
column-count: auto;
column-fill: balance;
column-gap: normal;
column-rule-color: currentcolor;
column-rule-style: none;
column-rule-width: medium;
column-span: none;
column-width: auto;
content: normal;
counter-increment: none;
counter-reset: none;
cursor: auto;
display: inline;
empty-cells: show;
filter: none;
flex-basis: auto;
flex-direction: row;
flex-grow: 0;
flex-shrink: 1;
flex-wrap: nowrap;
float: none;
font-family: initial;
font-feature-settings: normal;
font-kerning: auto;
font-language-override: normal;
font-size: medium;
font-size-adjust: none;
font-stretch: normal;
font-style: normal;
font-synthesis: weight style;
font-variant: normal;
font-variant-alternates: normal;
font-variant-caps: normal;
font-variant-east-asian: normal;
font-variant-ligatures: normal;
font-variant-numeric: normal;
font-variant-position: normal;
font-weight: 400;
grid-auto-columns: auto;
grid-auto-flow: row;
grid-auto-rows: auto;
grid-column-end: auto;
grid-column-gap: 0;
grid-column-start: auto;
grid-row-end: auto;
grid-row-gap: 0;
grid-row-start: auto;
grid-template-areas: none;
grid-template-columns: none;
grid-template-rows: none;
height: auto;
hyphens: manual;
image-orientation: 0deg;
image-rendering: auto;
image-resolution: 1dppx;
ime-mode: auto;
inline-size: auto;
isolation: auto;
justify-content: flexStart;
left: auto;
letter-spacing: normal;
line-break: auto;
line-height: normal;
list-style-image: none;
list-style-position: outside;
list-style-type: disc;
margin-block-end: 0;
margin-block-start: 0;
margin-bottom: 0;
margin-inline-end: 0;
margin-inline-start: 0;
margin-left: 0;
margin-right: 0;
margin-top: 0;
mask-clip: borderBox;
mask-composite: add;
mask-image: none;
mask-mode: matchSource;
mask-origin: borderBox;
mask-position: 0 0;
mask-repeat: repeat;
mask-size: auto;
mask-type: luminance;
max-height: none;
max-width: none;
min-block-size: 0;
min-height: 0;
min-inline-size: 0;
min-width: 0;
mix-blend-mode: normal;
object-fit: fill;
object-position: 50% 50%;
offset-block-end: auto;
offset-block-start: auto;
offset-inline-end: auto;
offset-inline-start: auto;
opacity: 1;
order: 0;
orphans: 2;
outline-color: initial;
outline-offset: 0;
outline-style: none;
outline-width: medium;
overflow: visible;
overflow-wrap: normal;
overflow-x: visible;
overflow-y: visible;
padding-block-end: 0;
padding-block-start: 0;
padding-bottom: 0;
padding-inline-end: 0;
padding-inline-start: 0;
padding-left: 0;
padding-right: 0;
padding-top: 0;
page-break-after: auto;
page-break-before: auto;
page-break-inside: auto;
perspective: none;
perspective-origin: 50% 50%;
pointer-events: auto;
position: static;
quotes: initial;
resize: none;
right: auto;
ruby-align: spaceAround;
ruby-merge: separate;
ruby-position: over;
scroll-behavior: auto;
scroll-snap-coordinate: none;
scroll-snap-destination: 0 0;
scroll-snap-points-x: none;
scroll-snap-points-y: none;
scroll-snap-type: none;
shape-image-threshold: 0;
shape-margin: 0;
shape-outside: none;
tab-size: 8;
table-layout: auto;
text-align: initial;
text-align-last: auto;
text-combine-upright: none;
text-decoration-color: currentcolor;
text-decoration-line: none;
text-decoration-style: solid;
text-emphasis-color: currentcolor;
text-emphasis-position: over right;
text-emphasis-style: none;
text-indent: 0;
text-justify: auto;
text-orientation: mixed;
text-overflow: clip;
text-rendering: auto;
text-shadow: none;
text-transform: none;
text-underline-position: auto;
top: auto;
touch-action: auto;
transform: none;
transform-box: borderBox;
transform-origin: 50% 50%0;
transform-style: flat;
transition-delay: 0s;
transition-duration: 0s;
transition-property: all;
transition-timing-function: ease;
vertical-align: baseline;
visibility: visible;
white-space: normal;
widows: 2;
width: auto;
will-change: auto;
word-break: normal;
word-spacing: normal;
word-wrap: normal;
writing-mode: horizontalTb;
z-index: auto;
-webkit-appearance: none;
-moz-appearance: none;
-ms-appearance: none;
appearance: none;
margin: 0;
}
.LiveAreaSection {
width: 100%;
}
.LiveAreaSection .login-option-buybox {
display: block;
width: 100%;
font-size: 17px;
line-height: 30px;
color: #222;
padding-top: 30px;
font-family: Harding, Palatino, serif;
}
.LiveAreaSection .additional-access-options {
display: block;
font-weight: 700;
font-size: 17px;
line-height: 30px;
color: #222;
font-family: Harding, Palatino, serif;
}
.LiveAreaSection .additional-login > li:not(:first-child)::before {
transform: translateY(-50%);
content: “”;
height: 1rem;
position: absolute;
top: 50%;
left: 0;
border-left: 2px solid #999;
}
.LiveAreaSection .additional-login > li:not(:first-child) {
padding-left: 10px;
}
.LiveAreaSection .additional-login > li {
display: inline-block;
position: relative;
vertical-align: middle;
padding-right: 10px;
}
.BuyBoxSection {
display: flex;
flex-wrap: wrap;
flex: 1;
flex-direction: row-reverse;
margin: -30px -15px 0;
}
.BuyBoxSection .box-inner {
width: 100%;
height: 100%;
padding: 30px 5px;
display: flex;
flex-direction: column;
justify-content: space-between;
}
.BuyBoxSection p {
margin: 0;
}
.BuyBoxSection .readcube-buybox {
background-color: #f3f3f3;
flex-shrink: 1;
flex-grow: 1;
flex-basis: 255px;
background-clip: content-box;
padding: 0 15px;
margin-top: 30px;
}
.BuyBoxSection .subscribe-buybox {
background-color: #f3f3f3;
flex-shrink: 1;
flex-grow: 4;
flex-basis: 300px;
background-clip: content-box;
padding: 0 15px;
margin-top: 30px;
}
.BuyBoxSection .subscribe-buybox-nature-plus {
background-color: #f3f3f3;
flex-shrink: 1;
flex-grow: 4;
flex-basis: 100%;
background-clip: content-box;
padding: 0 15px;
margin-top: 30px;
}
.BuyBoxSection .title-readcube,
.BuyBoxSection .title-buybox {
display: block;
margin: 0;
margin-right: 10%;
margin-left: 10%;
font-size: 24px;
line-height: 32px;
color: #222;
text-align: center;
font-family: Harding, Palatino, serif;
}
.BuyBoxSection .title-asia-buybox {
display: block;
margin: 0;
margin-right: 5%;
margin-left: 5%;
font-size: 24px;
line-height: 32px;
color: #222;
text-align: center;
font-family: Harding, Palatino, serif;
}
.BuyBoxSection .asia-link,
.Link-328123652,
.Link-2926870917,
.Link-2291679238,
.Link-595459207 {
color: #069;
cursor: pointer;
text-decoration: none;
font-size: 1.05em;
font-family: -apple-system, BlinkMacSystemFont, “Segoe UI”, Roboto,
Oxygen-Sans, Ubuntu, Cantarell, “Helvetica Neue”, sans-serif;
line-height: 1.05em6;
}
.BuyBoxSection .access-readcube {
display: block;
margin: 0;
margin-right: 10%;
margin-left: 10%;
font-size: 14px;
color: #222;
padding-top: 10px;
text-align: center;
font-family: -apple-system, BlinkMacSystemFont, “Segoe UI”, Roboto,
Oxygen-Sans, Ubuntu, Cantarell, “Helvetica Neue”, sans-serif;
line-height: 20px;
}
.BuyBoxSection ul {
margin: 0;
}
.BuyBoxSection .link-usp {
display: list-item;
margin: 0;
margin-left: 20px;
padding-top: 6px;
list-style-position: inside;
}
.BuyBoxSection .link-usp span {
font-size: 14px;
color: #222;
font-family: -apple-system, BlinkMacSystemFont, “Segoe UI”, Roboto,
Oxygen-Sans, Ubuntu, Cantarell, “Helvetica Neue”, sans-serif;
line-height: 20px;
}
.BuyBoxSection .access-asia-buybox {
display: block;
margin: 0;
margin-right: 5%;
margin-left: 5%;
font-size: 14px;
color: #222;
padding-top: 10px;
text-align: center;
font-family: -apple-system, BlinkMacSystemFont, “Segoe UI”, Roboto,
Oxygen-Sans, Ubuntu, Cantarell, “Helvetica Neue”, sans-serif;
line-height: 20px;
}
.BuyBoxSection .access-buybox {
display: block;
margin: 0;
margin-right: 10%;
margin-left: 10%;
font-size: 14px;
color: #222;
opacity: 0.8px;
padding-top: 10px;
text-align: center;
font-family: -apple-system, BlinkMacSystemFont, “Segoe UI”, Roboto,
Oxygen-Sans, Ubuntu, Cantarell, “Helvetica Neue”, sans-serif;
line-height: 20px;
}
.BuyBoxSection .price-buybox {
display: block;
font-size: 30px;
color: #222;
font-family: -apple-system, BlinkMacSystemFont, “Segoe UI”, Roboto,
Oxygen-Sans, Ubuntu, Cantarell, “Helvetica Neue”, sans-serif;
padding-top: 30px;
text-align: center;
}
.BuyBoxSection .price-buybox-to {
display: block;
font-size: 30px;
color: #222;
font-family: -apple-system, BlinkMacSystemFont, “Segoe UI”, Roboto,
Oxygen-Sans, Ubuntu, Cantarell, “Helvetica Neue”, sans-serif;
text-align: center;
}
.BuyBoxSection .price-info-text {
font-size: 16px;
padding-right: 10px;
color: #222;
font-family: -apple-system, BlinkMacSystemFont, “Segoe UI”, Roboto,
Oxygen-Sans, Ubuntu, Cantarell, “Helvetica Neue”, sans-serif;
}
.BuyBoxSection .price-value {
font-size: 30px;
font-family: -apple-system, BlinkMacSystemFont, “Segoe UI”, Roboto,
Oxygen-Sans, Ubuntu, Cantarell, “Helvetica Neue”, sans-serif;
}
.BuyBoxSection .price-per-period {
font-family: -apple-system, BlinkMacSystemFont, “Segoe UI”, Roboto,
Oxygen-Sans, Ubuntu, Cantarell, “Helvetica Neue”, sans-serif;
}
.BuyBoxSection .price-from {
font-size: 14px;
padding-right: 10px;
color: #222;
font-family: -apple-system, BlinkMacSystemFont, “Segoe UI”, Roboto,
Oxygen-Sans, Ubuntu, Cantarell, “Helvetica Neue”, sans-serif;
line-height: 20px;
}
.BuyBoxSection .issue-buybox {
display: block;
font-size: 13px;
text-align: center;
color: #222;
font-family: -apple-system, BlinkMacSystemFont, “Segoe UI”, Roboto,
Oxygen-Sans, Ubuntu, Cantarell, “Helvetica Neue”, sans-serif;
line-height: 19px;
}
.BuyBoxSection .no-price-buybox {
display: block;
font-size: 13px;
line-height: 18px;
text-align: center;
padding-right: 10%;
padding-left: 10%;
padding-bottom: 20px;
padding-top: 30px;
color: #222;
font-family: -apple-system, BlinkMacSystemFont, “Segoe UI”, Roboto,
Oxygen-Sans, Ubuntu, Cantarell, “Helvetica Neue”, sans-serif;
}
.BuyBoxSection .vat-buybox {
display: block;
margin-top: 5px;
margin-right: 20%;
margin-left: 20%;
font-size: 11px;
color: #222;
padding-top: 10px;
padding-bottom: 15px;
text-align: center;
font-family: -apple-system, BlinkMacSystemFont, “Segoe UI”, Roboto,
Oxygen-Sans, Ubuntu, Cantarell, “Helvetica Neue”, sans-serif;
line-height: 17px;
}
.BuyBoxSection .tax-buybox {
display: block;
width: 100%;
color: #222;
padding: 20px 16px;
text-align: center;
font-family: -apple-system, BlinkMacSystemFont, “Segoe UI”, Roboto,
Oxygen-Sans, Ubuntu, Cantarell, “Helvetica Neue”, sans-serif;
line-height: NaNpx;
}
.BuyBoxSection .button-container {
display: flex;
padding-right: 20px;
padding-left: 20px;
justify-content: center;
}
.BuyBoxSection .button-container > * {
flex: 1px;
}
.BuyBoxSection .button-container > a:hover,
.Button-505204839:hover,
.Button-1078489254:hover,
.Button-2737859108:hover {
text-decoration: none;
}
.BuyBoxSection .btn-secondary {
background: #fff;
}
.BuyBoxSection .button-asia {
background: #069;
border: 1px solid #069;
border-radius: 0;
cursor: pointer;
display: block;
padding: 9px;
outline: 0;
text-align: center;
text-decoration: none;
min-width: 80px;
margin-top: 75px;
}
.BuyBoxSection .button-label-asia,
.ButtonLabel-3869432492,
.ButtonLabel-3296148077,
.ButtonLabel-1636778223 {
display: block;
color: #fff;
font-size: 17px;
line-height: 20px;
font-family: -apple-system, BlinkMacSystemFont, “Segoe UI”, Roboto,
Oxygen-Sans, Ubuntu, Cantarell, “Helvetica Neue”, sans-serif;
text-align: center;
text-decoration: none;
cursor: pointer;
}
.Button-505204839,
.Button-1078489254,
.Button-2737859108 {
background: #069;
border: 1px solid #069;
border-radius: 0;
cursor: pointer;
display: block;
padding: 9px;
outline: 0;
text-align: center;
text-decoration: none;
min-width: 80px;
max-width: 320px;
margin-top: 20px;
}
.Button-505204839 .btn-secondary-label,
.Button-1078489254 .btn-secondary-label,
.Button-2737859108 .btn-secondary-label {
color: #069;
}
.uList-2102244549 {
list-style: none;
padding: 0;
margin: 0;
}
/* style specs end */
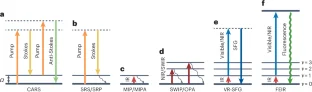
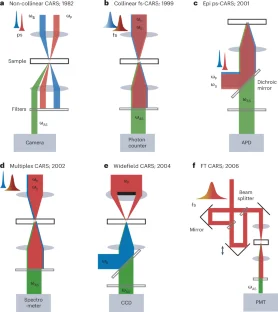
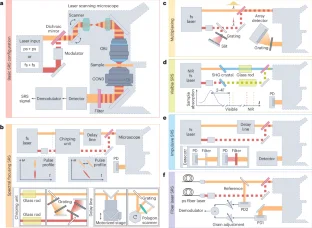
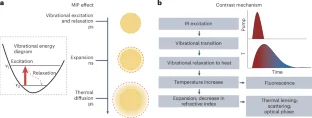
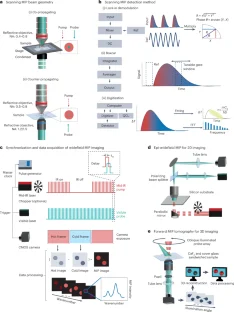
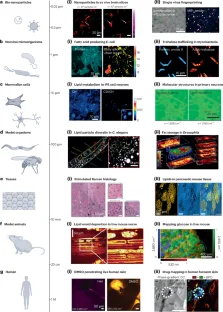
References
-
Duncan, M. D., Reintjes, J. & Manuccia, T. J. Scanning coherent anti-Stokes Raman microscope. Opt. Lett. 7, 350–352 (1982). CARS microscope using a non-collinear beam geometry.
Google Scholar
-
Zumbusch, A., Holtom, G. R. & Xie, X. S. Three-dimensional vibrational imaging by coherent anti-Stokes Raman scattering. Phys. Rev. Lett. 82, 4142–4145 (1999). Collinear CARS microscope. This paper revived the CARS microscopy field.
Google Scholar
-
Cheng, J. X., Volkmer, A., Book, L. D. & Xie, X. S. An epi-detected coherent anti-stokes raman scattering (E-CARS) microscope with high spectral resolution and high sensitivity. J. Phys. Chem. B 105, 1277–1280 (2001). Epi-detected CARS microscope.
Google Scholar
-
Evans, C. L. et al. Chemical imaging of tissue in vivo with video-rate coherent anti-Stokes Raman scattering microscopy. Proc. Natl Acad. Sci. USA 102, 16807–16812 (2005).
Google Scholar
-
Heuke, S. & Rigneault, H. Coherent Stokes Raman scattering microscopy (CSRS). Nat. Commun. 14, 3337 (2023).
Google Scholar
-
Cheng, J. X., Volkmer, A., Book, L. D. & Xie, X. S. Multiplex coherent anti-stokes Raman scattering microspectroscopy and study of lipid vesicles. J. Phys. Chem. B 106, 8493–8498 (2002).
Google Scholar
-
Wurpel, G. W., Schins, J. M. & Muller, M. Chemical specificity in three-dimensional imaging with multiplex coherent anti-Stokes Raman scattering microscopy. Opt. Lett. 27, 1093–1095 (2002).
Google Scholar
-
Kee, T. W. & Cicerone, M. T. Simple approach to one-laser, broadband coherent anti-Stokes Raman scattering microscopy. Opt. Lett. 29, 2701–2703 (2004).
Google Scholar
-
Camp Jr, C. H. et al. High-speed coherent Raman fingerprint imaging of biological tissues. Nat. Photonics 8, 627–634 (2014). Broadband CARS with high sensitivity.
Google Scholar
-
Volkmer, A., Book, L. D. & Xie, X. S. Time-resolved coherent anti-Stokes Raman scattering microscopy: imaging based on Raman free induction decay. Appl. Phys. Lett. 80, 1505–1507 (2002).
Google Scholar
-
Hellerer, T., Enejder, A. M. K. & Zumbusch, A. Spectral focusing: high spectral resolution spectroscopy with broad-bandwidth laser pulses. Appl. Phys. Lett. 85, 25–27 (2004).
Google Scholar
-
Ogilvie, J. P., Beaurepaire, E., Alexandrou, A. & Joffre, M. Fourier-transform coherent anti-Stokes Raman scattering microscopy. Opt. Lett. 31, 480–482 (2006).
Google Scholar
-
Ideguchi, T. et al. Coherent Raman spectro-imaging with laser frequency combs. Nature 502, 355–358 (2013).
Google Scholar
-
Hashimoto, K., Takahashi, M., Ideguchi, T. & Goda, K. Broadband coherent Raman spectroscopy running at 24,000 spectra per second. Sci. Rep. 6, 21036 (2016).
Google Scholar
-
Kinegawa, R. et al. High-speed broadband Fourier-transform coherent anti-stokes Raman scattering spectral microscopy. J. Raman Spectrosc. 50, 1141–1146 (2019).
Google Scholar
-
Heinrich, C., Bernet, S. & Ritsch-Marte, M. Wide-field coherent anti-Stokes Raman scattering microscopy. Appl. Phys. Lett. 84, 816–818 (2004).
Google Scholar
-
Toytman, I., Cohn, K., Smith, T., Simanovskii, D. & Palanker, D. Wide-field coherent anti-Stokes Raman scattering microscopy with non-phase-matching illumination. Opt. Lett. 32, 1941–1943 (2007).
Google Scholar
-
Heinrich, C., Hofer, A., Bernet, S. & Ritsch-Marte, M. Coherent anti-Stokes Raman scattering microscopy with dynamic speckle illumination. N. J. Phys. 10, 023029 (2008).
Google Scholar
-
Shi, K., Li, H., Xu, Q., Psaltis, D. & Liu, Z. Coherent anti-stokes Raman holography for chemically selective single-shot nonscanning 3D imaging. Phys. Rev. Lett. 104, 093902 (2010).
Google Scholar
-
Lei, M., Winterhalder, M., Selm, R. & Zumbusch, A. Video-rate wide-field coherent anti-Stokes Raman scattering microscopy with collinear nonphase-matching illumination. J. Biomed. Opt. 16, 021102 (2011).
Google Scholar
-
Berto, P., Gachet, D., Bon, P., Monneret, S. & Rigneault, H. Wide-field vibrational phase imaging. Phys. Rev. Lett. 109, 093902 (2012).
Google Scholar
-
Zong, C. et al. Wide-field surface-enhanced coherent anti-Stokes Raman scattering microscopy. ACS Photonics 9, 1042–1049 (2022).
Google Scholar
-
Fantuzzi, E. M. et al. Wide-field coherent anti-Stokes Raman scattering microscopy using random illuminations. Nat. Photonics 17, 1097–1104 (2023).
Google Scholar
-
Ploetz, E., Laimgruber, S., Berner, S., Zinth, W. & Gilch, P. Femtosecond stimulated Raman microscopy. Appl. Phys. B 87, 389–393 (2007). SRS microscope.
Google Scholar
-
Freudiger, C. W. et al. Label-free biomedical imaging with high sensitivity by stimulated Raman scattering microscopy. Science 322, 1857–1861 (2008). High-speed SRS microscope achieving high-sensitivity biomedical imaging with chemical specificity.
Google Scholar
-
Nandakumar, P., Kovalev, A. & Volkmer, A. Vibrational imaging based on stimulated Raman scattering microscopy. N. J. Phys. 11, 033026 (2009).
Google Scholar
-
Ozeki, Y., Dake, F., Kajiyama, S. I., Fukui, K. & Itoh, K. Analysis and experimental assessment of the sensitivity of stimulated Raman scattering microscopy. Opt. Express 17, 3651–3658 (2009).
Google Scholar
-
Saar, B. G. et al. Video-rate molecular imaging in vivo with stimulated Raman scattering. Science 330, 1368–1370 (2010).
Google Scholar
-
Li, H. et al. Imaging chemical kinetics of radical polymerization with an ultrafast coherent Raman microscope. Adv. Sci. 7, 1903644 (2020).
Google Scholar
-
Andresen, E. R., Berto, P. & Rigneault, H. Stimulated Raman scattering microscopy by spectral focusing and fiber-generated soliton as Stokes pulse. Opt. Lett. 36, 2387–2389 (2011).
Google Scholar
-
Beier, H. T., Noojin, G. D. & Rockwell, B. A. Stimulated Raman scattering using a single femtosecond oscillator with flexibility for imaging and spectral applications. Opt. Express 19, 18885–18892 (2011).
Google Scholar
-
Liao, C.-S. et al. Stimulated Raman spectroscopic imaging by microsecond delay-line tuning. Optica 3, 1377–1380 (2016).
Google Scholar
-
Lin, H. et al. Microsecond fingerprint stimulated Raman spectroscopic imaging by ultrafast tuning and spatial-spectral learning. Nat. Commun. 12, 3052 (2021).
Google Scholar
-
Alshaykh, M. S. et al. High-speed stimulated hyperspectral Raman imaging using rapid acousto-optic delay lines. Opt. Lett. 42, 1548–1551 (2017).
Google Scholar
-
Liao, C. S. et al. Microsecond scale vibrational spectroscopic imaging by multiplex stimulated Raman scattering microscopy. Light Sci. Appl. 4, e265 (2015).
Google Scholar
-
Fu, D. et al. Quantitative chemical imaging with multiplex stimulated Raman scattering microscopy. J. Am. Chem. Soc. 134, 3623–3626 (2012).
Google Scholar
-
Suzuki, Y. et al. Label-free chemical imaging flow cytometry by high-speed multicolor stimulated Raman scattering. Proc. Natl Acad. Sci. USA 116, 15842–15848 (2019).
Google Scholar
-
Karpf, S., Eibl, M., Wieser, W., Klein, T. & Huber, R. A time-encoded technique for fibre-based hyperspectral broadband stimulated Raman microscopy. Nat. Commun. 6, 6784 (2015).
Google Scholar
-
Ozeki, Y. et al. High-speed molecular spectral imaging of tissue with stimulated Raman scattering. Nat. Photonics 6, 844–850 (2012).
Google Scholar
-
Würthwein, T. et al. Multi-color stimulated Raman scattering with a frame-to-frame wavelength-tunable fiber-based light source. Biomed. Opt. Express 12, 6228–6236 (2021).
Google Scholar
-
Wei, L. et al. Super-multiplex vibrational imaging. Nature 544, 465–470 (2017).
Google Scholar
-
Xiong, H. et al. Stimulated Raman excited fluorescence spectroscopy and imaging. Nat. Photonics 13, 412–417 (2019).
Google Scholar
-
Xiong, H. et al. Super-resolution vibrational microscopy by stimulated Raman excited fluorescence. Light Sci. Appl. 10, 87 (2021).
Google Scholar
-
Ao, J. et al. Switchable stimulated Raman scattering microscopy with photochromic vibrational probes. Nat. Commun. 12, 3089 (2021).
Google Scholar
-
Shou, J. et al. Super-resolution vibrational imaging based on photoswitchable Raman probe. Sci. Adv. 9, eade9118 (2023).
Google Scholar
-
Ao, J. et al. Photoswitchable vibrational nanoscopy with sub-100-nm optical resolution. Adv. Photonics 5, 066001 (2023).
Google Scholar
-
Zong, C. et al. Plasmon-enhanced stimulated Raman scattering microscopy with single-molecule detection sensitivity. Nat. Commun. 10, 5318 (2019).
Google Scholar
-
Zhuge, M. et al. Ultrasensitive vibrational imaging of retinoids by visible preresonance stimulated Raman scattering microscopy. Adv. Sci. 8, 2003136 (2021).
Google Scholar
-
Bi, Y. et al. Near-resonance enhanced label-free stimulated Raman scattering microscopy with spatial resolution near 130 nm. Light Sci. Appl. 7, 81 (2018).
Google Scholar
-
Koehl, R. M., Adachi, S. & Nelson, K. A. Real-space polariton wave packet imaging. J. Chem. Phys. 110, 1317–1320 (1999).
Google Scholar
-
Raanan, D. et al. Sub-second hyper-spectral low-frequency vibrational imaging via impulsive Raman excitation. Opt. Lett. 44, 5153–5156 (2019).
Google Scholar
-
Chen, X. et al. Volumetric chemical imaging by stimulated Raman projection microscopy and tomography. Nat. Commun. 8, 15117 (2017).
Google Scholar
-
Wang, W. & Huang, Z. Stimulated Raman scattering tomography for rapid three-dimensional chemical imaging of cells and tissue. Adv. Photonics 6, 026001 (2024).
Google Scholar
-
Robles, F. E., Zhou, K. C., Fischer, M. C. & Warren, W. S. Stimulated Raman scattering spectroscopic optical coherence tomography. Optica 4, 243–246 (2017).
Google Scholar
-
Lin, P. et al. Volumetric chemical imaging in vivo by a remote-focusing stimulated Raman scattering microscope. Opt. Express 28, 30210–30221 (2020).
Google Scholar
-
Nose, K. et al. Sensitivity enhancement of fiber-laser-based stimulated Raman scattering microscopy by collinear balanced detection technique. Opt. Express 20, 13958–13965 (2012).
Google Scholar
-
Freudiger, C. W. et al. Stimulated Raman scattering microscopy with a robust fibre laser source. Nat. Photonics 8, 153–159 (2014).
Google Scholar
-
Crisafi, F. et al. In-line balanced detection stimulated Raman scattering microscopy. Sci. Rep. 7, 10745 (2017).
Google Scholar
-
Orringer, D. A. et al. Rapid intraoperative histology of unprocessed surgical specimens via fibre-laser-based stimulated Raman scattering microscopy. Nat. Biomed. Eng. 1, 0027 (2017). Clinical translation of stimulated Raman histology for intraoperative use.
Google Scholar
-
Liao, C.-S. et al. In vivo and in situ spectroscopic imaging by a handheld stimulated Raman scattering microscope. ACS Photonics 5, 947–954 (2017).
Google Scholar
-
Lin, H. & Cheng, J. X. Computational coherent Raman scattering imaging: breaking physical barriers by fusion of advanced instrumentation and data science. eLight 3, 6 (2023).
Google Scholar
-
Zhang, D. et al. Depth-resolved mid-infrared photothermal imaging of living cells and organisms with submicrometer spatial resolution. Sci. Adv. 2, e1600521 (2016). MIP imaging of living systems. This paper led to mIRage, a commercial product that is worldwide used.
-
Li, Z., Aleshire, K., Kuno, M. & Hartland, G. V. Super-resolution far-field infrared imaging by photothermal heterodyne imaging. J. Phys. Chem. B 121, 8838–8846 (2017).
Google Scholar
-
Dong, P. T. et al. Polarization-sensitive stimulated Raman scattering imaging resolves amphotericin B orientation in Candida membrane. Sci. Adv. 7, eabd5230 (2021).
Google Scholar
-
Yurdakul, C., Zong, H. N., Bai, Y. R., Cheng, J. X. & Ünlü, M. S. Bond-selective interferometric scattering microscopy. J. Phys. D. 54, 364002 (2021).
Google Scholar
-
Xia, Q. et al. Single virus fingerprinting by widefield interferometric defocus-enhanced mid-infrared photothermal microscopy. Nat. Commun. 14, 6655 (2023).
Google Scholar
-
Yuan, T., Riobo, L., Gasparin, F., Ntziachristos, V. & Pleitez, M. A. Phase-shifting optothermal microscopy enables live-cell mid-infrared hyperspectral imaging of large cell populations at high confluency. Sci. Adv. 10, eadj7944 (2024).
Google Scholar
-
Zhang, D. et al. Bond-selective transient phase imaging via sensing of the infrared photothermal effect. Light Sci. Appl. 8, 116 (2019).
Google Scholar
-
Schnell, M. et al. All-digital histopathology by infrared-optical hybrid microscopy. Proc. Natl Acad. Sci. USA 117, 3388–3396 (2020).
Google Scholar
-
Toda, K., Tamamitsu, M. & Ideguchi, T. Adaptive dynamic range shift (ADRIFT) quantitative phase imaging. Light Sci. Appl. 10, 1 (2021).
Google Scholar
-
Zhang, Y. et al. Fluorescence-detected mid-infrared photothermal microscopy. J. Am. Chem. Soc. 143, 11490–11499 (2021).
Google Scholar
-
Li, M. et al. Fluorescence-detected mid-infrared photothermal microscopy. J. Am. Chem. Soc. 143, 10809–10815 (2021).
Google Scholar
-
Yin, J. et al. Video-rate mid-infrared photothermal imaging by single-pulse photothermal detection per pixel. Sci. Adv. 9, eadg8814 (2023).
Google Scholar
-
He, H. et al. Mapping enzyme activity in living systems by real-time mid-infrared photothermal imaging of nitrile chameleons. Nat. Methods 21, 342–352 (2024).
Google Scholar
-
Adhikari, S. et al. Photothermal microscopy: imaging the optical absorption of single nanoparticles and single molecules. ACS Nano 14, 16414–16445 (2020).
Google Scholar
-
Samolis, P. D. & Sander, M. Y. Phase-sensitive lock-in detection for high-contrast mid-infrared photothermal imaging with sub-diffraction limited resolution. Opt. Express 27, 2643–2655 (2019).
Google Scholar
-
Yin, J. et al. Nanosecond-resolution photothermal dynamic imaging via MHZ digitization and match filtering. Nat. Commun. 12, 7097 (2021).
Google Scholar
-
Samolis, P. D., Zhu, X. & Sander, M. Y. Time-resolved mid-infrared photothermal microscopy for imaging water-embedded axon bundles. Anal. Chem. 95, 16514–16521 (2023).
Google Scholar
-
Bai, Y. et al. Ultrafast chemical imaging by widefield photothermal sensing of infrared absorption. Sci. Adv. 5, eaav7127 (2019).
Google Scholar
-
Toda, K., Tamamitsu, M., Nagashima, Y., Horisaki, R. & Ideguchi, T. Molecular contrast on phase-contrast microscope. Sci. Rep. 9, 9957 (2019).
Google Scholar
-
Ishigane, G. et al. Label-free mid-infrared photothermal live-cell imaging beyond video rate. Light Sci. Appl. 12, 174 (2023).
Google Scholar
-
Tamamitsu, M. et al. Label-free biochemical quantitative phase imaging with mid-infrared photothermal effect. Optica 7, 359–366 (2020).
Google Scholar
-
Zhao, J. et al. Bond-selective intensity diffraction tomography. Nat. Commun. 13, 7767 (2022).
Google Scholar
-
Zhu, Y. et al. Stimulated Raman photothermal microscopy toward ultrasensitive chemical imaging. Sci. Adv. 9, eadi2181 (2023). Stimulated Raman photothermal microscope.
Google Scholar
-
Wilson, R. H., Nadeau, K. P., Jaworski, F. B., Tromberg, B. J. & Durkin, A. J. Review of short-wave infrared spectroscopy and imaging methods for biological tissue characterization. J. Biomed. Opt. 20, 030901 (2015).
Google Scholar
-
Wang, P., Rajian, J. R. & Cheng, J. X. Spectroscopic imaging of deep tissue through photoacoustic detection of molecular vibration. J. Phys. Chem. Lett. 4, 2177–2185 (2013).
Google Scholar
-
Ni, H. et al. Millimetre-deep micrometre-resolution vibrational imaging by shortwave infrared photothermal microscopy. Nat. Photonics 18, 944–951 (2024). Vibrational photothermal microscope in the short-wave infrared window enabling subcellular-resolution and millimeter-deep chemical imaging.
Google Scholar
-
Wang, L. et al. Overtone photothermal microscopy for high-resolution and high-sensitivity vibrational imaging. Nat. Commun. 15, 5374 (2024).
Google Scholar
-
Wang, H. W. et al. Label-free bond-selective imaging by listening to vibrationally excited molecules. Phys. Rev. Lett. 106, 238106 (2011). Bond-selective photoacoustic microscope.
Google Scholar
-
Horton, N. G. et al. In vivo three-photon microscopy of subcortical structures within an intact mouse brain. Nat. Photonics 7, 205–209 (2013).
Google Scholar
-
Wang, L. V. & Wu, H. I. Biomedical Optics: Principles and Imaging (John Wiley & Sons, 2012).
-
Sim, J. Y., Ahn, C. G., Jeong, E. J. & Kim, B. K. In vivo microscopic photoacoustic spectroscopy for non-invasive glucose monitoring invulnerable to skin secretion products. Sci. Rep. 8, 1059 (2018).
Google Scholar
-
Pleitez, M. A. et al. Label-free metabolic imaging by mid-infrared optoacoustic microscopy in living cells. Nat. Biotechnol. 38, 293–296 (2020).
Google Scholar
-
Uluc, N. et al. Non-invasive measurements of blood glucose levels by time-gating mid-infrared optoacoustic signals. Nat. Metab. 6, 678–686 (2024).
Google Scholar
-
Shi, J. et al. High-resolution, high-contrast mid-infrared imaging of fresh biological samples with ultraviolet-localized photoacoustic microscopy. Nat. Photonics 13, 609–615 (2019). MIPA microscope.
Google Scholar
-
Xu, Z., Zhu, Q. & Wang, L. V. In vivo photoacoustic tomography of mouse cerebral edema induced by cold injury. J. Biomed. Opt. 16, 066020 (2011).
Google Scholar
-
Jansen, K., van der Steen, A. F., van Beusekom, H. M., Oosterhuis, J. W. & van Soest, G. Intravascular photoacoustic imaging of human coronary atherosclerosis. Opt. Lett. 36, 597–599 (2011).
Google Scholar
-
Wang, P. et al. High-speed intravascular photoacoustic imaging of lipid-laden atherosclerotic plaque enabled by a 2-kHz barium nitrite raman laser. Sci. Rep. 4, 6889 (2014).
Google Scholar
-
Li, R. et al. High-speed intraoperative assessment of breast tumor margins by multimodal ultrasound and photoacoustic tomography. Med. Devices Sens. 1, e10018 (2018).
Google Scholar
-
Yakovlev, V. V. et al. Stimulated Raman photoacoustic imaging. Proc. Natl Acad. Sci. USA 107, 20335–20339 (2010).
Google Scholar
-
Raghunathan, V., Han, Y., Korth, O., Ge, N. H. & Potma, E. O. Rapid vibrational imaging with sum frequency generation microscopy. Opt. Lett. 36, 3891–3893 (2011).
Google Scholar
-
Hanninen, A. M., Prince, R. C., Ramos, R., Plikus, M. V. & Potma, E. O. High-resolution infrared imaging of biological samples with third-order sum-frequency generation microscopy. Biomed. Opt. Express 9, 4807–4817 (2018).
Google Scholar
-
Whaley-Mayda, L., Guha, A., Penwell, S. B. & Tokmakoff, A. Fluorescence-encoded infrared vibrational spectroscopy with single-molecule sensitivity. J. Am. Chem. Soc. 143, 3060–3064 (2021).
Google Scholar
-
Wang, H. et al. Bond-selective fluorescence imaging with single-molecule sensitivity. Nat. Photonics 17, 846–855 (2023).
Google Scholar
-
Yan, C. et al. Multidimensional widefield infrared-encoded spontaneous emission microscopy: distinguishing chromophores by ultrashort infrared pulses. J. Am. Chem. Soc. 146, 1874–1886 (2024).
Google Scholar
-
Vanden-Hehir, S. et al. Alkyne-tagged PLGA allows direct visualization of nanoparticles in vitro and ex vivo by stimulated Raman scattering microscopy. Biomacromolecules 20, 4008–4014 (2019).
Google Scholar
-
Xia, Q. et al. Click-free imaging of carbohydrate trafficking in live cells using an azido photothermal probe. Sci. Adv. 10, eadq0294 (2024).
Google Scholar
-
Tague, N. et al. Longitudinal single-cell imaging of engineered strains with stimulated Raman scattering to characterize heterogeneity in fatty acid production. Adv. Sci. 10, e2206519 (2023).
Google Scholar
-
Ge, X. et al. SRS-FISH: a high-throughput platform linking microbiome metabolism to identity at the single-cell level. Proc. Natl Acad. Sci. USA 119, e2203519119 (2022).
Google Scholar
-
Zhang, J. et al. Visualization of a limonene synthesis metabolon inside living bacteria by hyperspectral SRS microscopy. Adv. Sci. 9, e2203887 (2022).
Google Scholar
-
Wakisaka, Y. et al. Probing the metabolic heterogeneity of live Euglena gracilis with stimulated Raman scattering microscopy. Nat. Microbiol. 1, 16124 (2016).
Google Scholar
-
Schiessl, K. T. et al. Phenazine production promotes antibiotic tolerance and metabolic heterogeneity in Pseudomonas aeruginosa biofilms. Nat. Commun. 10, 762 (2019).
Google Scholar
-
Zhang, M. et al. Rapid determination of antimicrobial susceptibility by stimulated Raman scattering imaging of D2O metabolic incorporation in a single bacterium. Adv. Sci. 7, 2001452 (2020).
Google Scholar
-
Tan, Y., Lin, H. & Cheng, J. X. Profiling single cancer cell metabolism via high-content SRS imaging with chemical sparsity. Sci. Adv. 9, eadg6061 (2023).
Google Scholar
-
Zhao, G. et al. Ovarian cancer cell fate regulation by the dynamics between saturated and unsaturated fatty acids. Proc. Natl Acad. Sci. USA 119, e2203480119 (2022).
Google Scholar
-
Bai, Y. et al. Single-cell mapping of lipid metabolites using an infrared probe in human-derived model systems. Nat. Commun. 15, 350 (2024).
Google Scholar
-
Li, J. et al. Lipid desaturation is a metabolic marker and therapeutic target of ovarian cancer stem cells. Cell Stem Cell 20, 303–314 (2017).
Google Scholar
-
Zhang, X. et al. Label-free live-cell imaging of nucleic acids using stimulated Raman scattering microscopy. Chemphyschem 13, 1054–1059 (2012).
Google Scholar
-
Lu, F. K. et al. Label-free DNA imaging in vivo with stimulated Raman scattering microscopy. Proc. Natl Acad. Sci. USA 112, 11624–11629 (2015).
Google Scholar
-
Oh, S. et al. Protein and lipid mass concentration measurement in tissues by stimulated Raman scattering microscopy. Proc. Natl Acad. Sci. USA 119, e2117938119 (2022).
Google Scholar
-
Lim, J. M. et al. Cytoplasmic protein imaging with mid-infrared photothermal microscopy: cellular dynamics of live neurons and oligodendrocytes. J. Phys. Chem. Lett. 10, 2857–2861 (2019).
Google Scholar
-
Hu, F. et al. Vibrational imaging of glucose uptake activity in live cells and tissues by stimulated Raman scattering. Angew. Chem. Int. Ed. Engl. 54, 9821–9825 (2015).
Google Scholar
-
Li, Y. et al. Microglial lipid droplet accumulation in tauopathy brain is regulated by neuronal AMPK. Cell Metab. 36, 1351–1370 (2024).
Google Scholar
-
Gustavsson, N. et al. Correlative optical photothermal infrared and X-ray fluorescence for chemical imaging of trace elements and relevant molecular structures directly in neurons. Light Sci. Appl. 10, 151 (2021).
Google Scholar
-
Klementieva, O. et al. Super-resolution infrared imaging of polymorphic amyloid aggregates directly in neurons. Adv. Sci. 7, 1903004 (2020). Application of MIP microscopy to protein structure analysis.
Google Scholar
-
Wang, M. C., Min, W., Freudiger, C. W., Ruvkun, G. & Xie, X. S. RNAi screening for fat regulatory genes with SRS microscopy. Nat. Methods 8, 135–138 (2011).
Google Scholar
-
Wang, P. et al. Imaging lipid metabolism in live Caenorhabditis elegans using fingerprint vibrations. Angew. Chem. Int. Ed. Engl. 53, 11787–11792 (2014).
Google Scholar
-
Chen, W. W. et al. Spectroscopic coherent Raman imaging of Caenorhabditis elegans reveals lipid particle diversity. Nat. Chem. Biol. 16, 1087–1095 (2020).
Google Scholar
-
Li, Y., Zhang, W., Fung, A. A. & Shi, L. DO-SRS imaging of diet regulated metabolic activities in Drosophila during aging processes. Aging Cell 21, e13586 (2022).
Google Scholar
-
Hollon, T. C. et al. Near real-time intraoperative brain tumor diagnosis using stimulated Raman histology and deep neural networks. Nat. Med. 26, 52–58 (2020).
Google Scholar
-
Ji, M. et al. Label-free imaging of amyloid plaques in Alzheimer’s disease with stimulated Raman scattering microscopy. Sci. Adv. 4, eaat7715 (2018).
Google Scholar
-
Randall, E. C. et al. Integrated mapping of pharmacokinetics and pharmacodynamics in a patient-derived xenograft model of glioblastoma. Nat. Commun. 9, 4904 (2018).
Google Scholar
-
Ji, M. et al. Rapid, label-free detection of brain tumors with stimulated Raman scattering microscopy. Sci. Transl. Med. 5, 201ra119 (2013). Use of SRS microscopy for histopathology.
Google Scholar
-
Sethuraman, S., Amirian, J. H., Litovsky, S. H., Smalling, R. W. & Emelianov, S. Y. Ex vivo characterization of atherosclerosis using intravascular photoacoustic imaging. Opt. Express 15, 16657–16666 (2007).
Google Scholar
-
Wu, W., Wang, P., Cheng, J. X. & Xu, X. M. Assessment of white matter loss using bond-selective photoacoustic imaging in a rat model of contusive spinal cord injury. J. Neurotrauma 31, 1998–2002 (2014).
Google Scholar
-
Tian, F. et al. Monitoring peripheral nerve degeneration in ALS by label-free stimulated Raman scattering imaging. Nat. Commun. 7, 13283 (2016).
Google Scholar
-
Wang, B. et al. In vivo intravascular ultrasound-guided photoacoustic imaging of lipid in plaques using an animal model of atherosclerosis. Ultrasound Med. Biol. 38, 2098–2103 (2012).
Google Scholar
-
Li, M. et al. Ultrasensitive in vivo infrared spectroscopic imaging via oblique photothermal microscopy. Preprint at bioRxiv https://doi.org/10.1101/2024.10.02.616360 (2024).
-
Wei, L. et al. Live-cell imaging of alkyne-tagged small biomolecules by stimulated Raman scattering. Nat. Methods 11, 410–412 (2014).
Google Scholar
-
Shi, L. et al. Highly-multiplexed volumetric mapping with Raman dye imaging and tissue clearing. Nat. Biotechnol. 40, 364–373 (2022).
Google Scholar
-
Kamp, M. et al. Raman micro-spectroscopy reveals the spatial distribution of fumarate in cells and tissues. Nat. Commun. 15, 5386 (2024).
Google Scholar
-
Liu, X., Shi, L., Zhao, Z., Shu, J. & Min, W. VIBRANT: spectral profiling for single-cell drug responses. Nat. Methods 21, 501–511 (2024).
Google Scholar
-
Su, Y. et al. Steam disinfection releases micro(nano)plastics from silicone-rubber baby teats as examined by optical photothermal infrared microspectroscopy. Nat. Nanotechnol. 17, 76–85 (2022).
Google Scholar
-
Wei, M. et al. [Review on vibrational probes.] Nat. Methods https://doi.org/xxx (2025).
-
Fournier, D., Lepoutre, F. & Boccara, A. C. Tomographic approach for photothermal imaging using the mirage effect. J. Phys. Colloq. 44, C6-479–C476-482 (1983).
Google Scholar
-
Furstenberg, R, Kendziora, C. A., Papantonakis, M. R., Nguyen, V., & McGill, R. A. Chemical imaging using infrared photothermal microspectroscopy. in Proceedings of SPIE Defense, Security, and Sensing 8374, 837411 (2012).
-
Bai, Y., Yin, J. & Cheng, J. -X. Bond-selective imaging by optically sensing the mid-infrared photothermal effect. Sci. Adv. 7, eabg1559 (2021).
Google Scholar
-
Xia, Q., Yin, J., Guo, Z. & Cheng, J. -X. Mid-infrared photothermal microscopy: principle, instrumentation, applications. J. Phys. Chem. B 126, 8597–8613 (2022).
Google Scholar
-
Product news. Microscope improves infrared spectroscopy. J. Fail. Anal. Prev. 18, 250–251 (2018).
-
Fu, P. C. et al. Super-resolution imaging of non-fluorescent molecules by photothermal relaxation localization microscopy. Nat. Photonics 17, 330–337 (2023).
Google Scholar
-
Tamamitsu, M. et al. Mid-infrared wide-field nanoscopy. Nat. Photonics 18, 738–743 (2024).
Google Scholar
Acknowledgements
We acknowledge grants from the National Institutes of Health (R35 GM136223, R01 EB032391, R01 EB035429, R01 AI141439, R01 CA224275 and R33CA287046) and the Chan Zuckerberg Initiative DAF (2023-321163), an advised fund of Silicon Valley Community Foundation.
Author information
Authors and Affiliations
Contributions
J.-X.C. drafted the outline, introduction, outlook and two boxes. Y.Y. drafted the CARS section. H.N. drafted the SRS and SWIP sections. J.A. drafted the data science section. R.B. and Q.X. drafted the MIP section. X.G. and H.N. drafted the SRP and SWIP section. Q.X., J.A. and Y.Y. drafted the applications and user guide section. J.A., Q.X. and Y.Y. contributed to manuscript revision.
Corresponding author
Ethics declarations
Competing interests
J.-X.C. declares competing interests with VibroniX and Photothermal Spectroscopy, which did not fund this work. The remaining authors declare no competing interests.
Peer review
Peer review information
Nature Methods thanks Caitlin Davis, Vladislav Yakovlev, and the other, anonymous, reviewer(s) for their contribution to the peer review of this work. Primary Handling Editor: Rita Strack, in collaboration with the Nature Methods team.
Additional information
Publisher’s note Springer Nature remains neutral with regard to jurisdictional claims in published maps and institutional affiliations.
Supplementary information
Supplementary Information
Supplementary Notes and Supplementary Tables 1–5
Rights and permissions
Springer Nature or its licensor (e.g. a society or other partner) holds exclusive rights to this article under a publishing agreement with the author(s) or other rightsholder(s); author self-archiving of the accepted manuscript version of this article is solely governed by the terms of such publishing agreement and applicable law.
Reprints and permissions
About this article
Cite this article
Cheng, JX., Yuan, Y., Ni, H. et al. Advanced vibrational microscopes for life science.
Nat Methods 22, 912–927 (2025). https://doi.org/10.1038/s41592-025-02655-w
-
Received: 07 July 2024
-
Accepted: 04 March 2025
-
Published: 13 May 2025
-
Issue Date: May 2025
-
DOI: https://doi.org/10.1038/s41592-025-02655-w