Abstract
Human pressures have pushed the Earth system deep into the Anthropocene, threatening its stability, resilience and functioning. The Planetary Boundaries (PB) framework emerged against these threats, setting safe levels to the biophysical systems and processes that, with high likelihood, ensure life-supporting Holocene-like conditions. In this Review, we synthesize PB advancements, detailing its emergence and mainstreaming across scientific disciplines and society. The nine PBs capture the key functions regulating the Earth system. The safe operating space has been transgressed for six of these. PB science is essential to prevent further Earth system risks and has sparked new research on the precision of safe boundaries. Human development within planetary boundaries defines sustainable development, informing advances in social sciences. Each PB translates to a finite budget that the world must operate within, requiring strengthened global governance. The PB framework has been adopted by businesses and informed policy across the world, informing new thinking about fundamental justice concerns, and has inspired, among other concepts, the planetary commons, planetary health and doughnut economics. Future work must increase the precision and frequency of PB analyses, and, together with Earth observation data analytics, produce a high-resolution and real-time state of planetary health.
Similar content being viewed by others
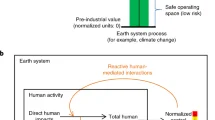
Human impacts on planetary boundaries amplified by Earth system interactions
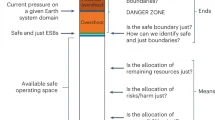
Earth system justice needed to identify and live within Earth system boundaries
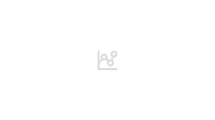
Planetary well-being
Introduction
Various socioeconomic and Earth system metrics rapidly and simultaneously surged around 1950, with this ‘great acceleration’ pushing humans into the Anthropocene1,2,3,4. There have since been increasing signs that Earth’s biogeochemical and physical capacities to maintain resilience are reaching saturation5. Tipping points are rapidly being approached, the crossing of which would cause often irreversible shifts in Earth system functioning6,7,8, threatening conditions for life as it is known5,9,10.
The Planetary Boundaries (PB) framework emerged from this knowledge11,12. The framework establishes global boundaries, which, if transgressed, substantially increase the risk of a change in Earth system functioning and/or its long-term state and trajectory13,14. Thus, it assesses the risk of steering the Earth system out from relatively stable15, favourable conditions and, in doing so, defines a safe operating space for humanity. The reference for a habitable planet is set to the only geological epoch known (for certain) to be able to support civilization establishment16 and human development17 — the Holocene, beginning ~11,700 years ago. Nine PBs are defined, covering the key geosphere and biosphere functions that regulate the state of the Earth system: climate change, introduction of novel entities, stratospheric ozone depletion, atmospheric aerosol loading, ocean acidification, biogeochemical flows, freshwater change, land system change, and change in biosphere integrity. Since the original formulation in 2009 (PB 1.0; refs. 11,12), the framework has been updated in 2015 (PB 2.0; ref. 18) and 2023 (PB 3.0; ref. 19). The latest iteration quantitatively assesses the status of all nine PBs for the first time.
The PB framework has seen widespread uptake (Fig. 1). Indeed, it has inspired countless scientific publications, and consequently, civil society initiatives, business practices and policy implementation20,21,22. For example, three PB articles (including two direct assessments11,18 and one informed by the PB framework23) appear in the top ten most cited scientific papers in global policy documents24. PBs have further given rise to broad societal discourse on action for global sustainability, sparking scientific debates about the environmental risks of anthropogenic pressures and the capacity of societies to navigate sustainable pathways25.
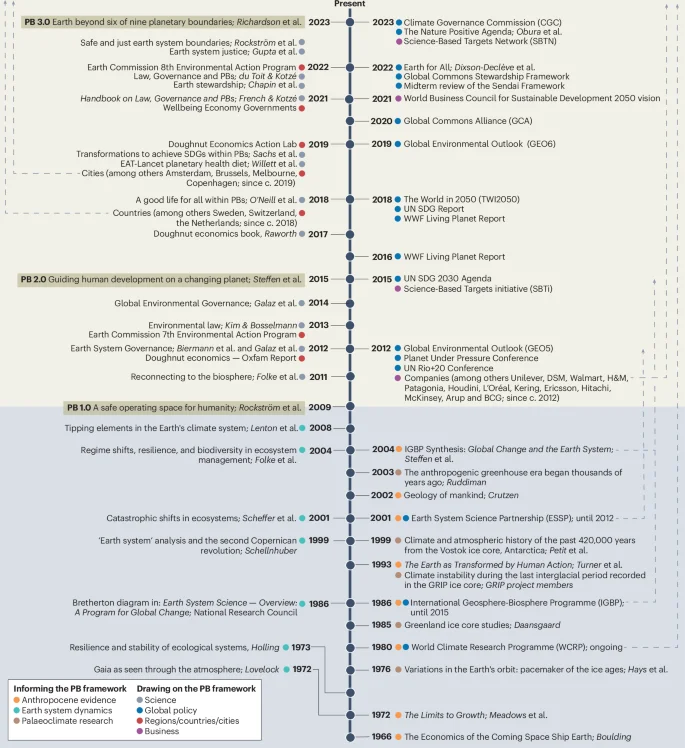
Key features informing (bottom) and drawing on (top) the Planetary Boundary (PB) framework, including science (grey), global policy (blue), regions/countries/cities (red) business (purple), Anthropocene evidence (orange), Earth system dynamics (teal) and palaeoclimate research (brown). PB science is not only informed by multiple strands of scientific enquiry but has influenced academia and policy across disciplines and sectors. SDG, Sustainable Development Goals.
In this Review, we take stock of the evolution, applications, and societal and policy impacts of the PB framework. We begin by discussing the emergence of the PB framework, including the identification of the nine PBs and their assessments. We follow with consideration of the broadscale adoption of the PB framework in Earth system science, governance, economics and sustainable development, and their integration. The use of PBs in society is subsequently discussed, focusing on their implementation for business and policy. We next examine the opportunities and challenges experienced in operationalizing the framework for navigating transformations to sustainable development in the Anthropocene. Finally, we reflect on the future development of the PB framework and science and their roles for furthering the global sustainability agenda.
Earth’s safe operating space
The PBs define the safe operating space for humanity. The framework’s emergence, evolution and analytical quantification are now discussed.
Emergence and fundamentals of the PB framework
The PB framework was originally presented as a ‘challenge’ to the scientific community26, asking for critical review of the idea. It was sparked by overwhelming evidence of the great acceleration3 and accompanying consensus that human activities threatened the functioning and state of the Earth system — humanity is now a dominant force of environmental change at the planetary scale, indicating the dawn of the Anthropocene2,27 (irrespective of the 2024 decision by stratigraphers not to call it a geological epoch28). The framework also arose from pioneering ecological–economic approaches29,30 such as Spaceship Earth31, Limits to Growth32 and Steady-State Economy33. Thus, the PB framework has multidisciplinary roots informed by Earth system dynamics34, palaeoclimate research35, and resilience and complex adaptive systems thinking36,37. These disciplines all highlighted Earth as a complex, partially self-regulating, geosphere–biosphere system38,39,40 with nonlinear behaviour and tipping points8,41 (Fig. 1) — a system where resilience and stability at planetary scale are determined by the dynamics of damping (negative) feedbacks and amplifying (positive) feedbacks.
In this way, the PB framework was a natural step in the integration of Earth system science and sustainability science34,42,43. It complemented other approaches of measuring and addressing sustainability challenges (such as the Ecological Footprint network44 or ecological carrying capacity), demonstrating the need to consider environmental challenges at the planetary scale. Relatedly, the emergence of PBs coincided with wider societal discourse on the need to identify guardrails or limits to human pressures on critical Earth system processes43.
Against this background, the fundamental ideas behind the PB framework took shape, identifying the processes and systems that regulate the Earth system and delineating a ‘safe operating space’ for humanity. Rather than focusing on a single dimension of the environmental crisis (such as anthropogenic climate change or pollution), the PB framework takes a systemic planetary perspective. For key control variables, boundaries are identified beyond which there is a substantially increased risk of changes in Earth system state and functionality. Importantly, these ‘boundaries’ are not synonyms for thresholds or tipping points. Instead, they are to be understood as biophysical boundaries45 that are informed by thresholds in some cases (as for climate change), and by gradually increasing risk gradients in others18 (such as land system change or freshwater change). It is a fundamental property of the PB framework that boundaries are set purely on biophysical grounds, without incorporating any consideration of specific human needs, feasibility or demands.
The space delineated by these boundaries is the ‘safe operating space for humanity’, named as such because stable Earth system state and functionality are preconditions for human development. The reference point for this safe operating space is the Holocene15,46, a climatically stable time period wherein global mean surface temperature oscillated ~14 °C ± 0.5 °C (ref. 15). Although modern Homo sapiens have existed on Earth for at least 250,000 years35,47,48, stable environmental conditions during the Holocene allowed humans to establish agriculture and sedentary communities49,50, initiating civilizations as they are now known. At present-day warming of 1.2 °C above preindustrial (the warmest temperature on Earth over the past 100,000 years51), this fundamental Holocene life-support is at risk. Importantly, the PB reference is ‘Holocene-like’ to clarify that boundaries are not set to theoretically return to a virgin Holocene state, but rather to maintain the Earth system within a (manageable) interglacial equilibrium state during the Anthropocene.
The Anthropocene and the PB framework both have origins in Earth system science — they go hand in hand. Together, they constitute potent drivers for integrating Earth system risks (risks to state and functioning of the Earth system) and the need for Earth system stewardship. Both provide the evidence (across all academic fields) that humanity needs to find ways of being stewards of its interactions with the planet as a whole to ensure its future. The need to raise stewardship to the planetary scale is not suggesting a top-down anthropocentric management of the planet, but it does suggest the need for humanity to reconnect the human world with planet Earth, and for all human interactions with the living and non-living parts of the planet to align with Earth stability and resilience.
Assessing Earth system risks with PBs
Nine systems and processes were selected to comprehensively and systematically capture the key functions that regulate the Earth system on its trajectory in the Anthropocene: climate change, introduction of novel entities, stratospheric ozone depletion, atmospheric aerosol loading, ocean acidification, biogeochemical flows, freshwater change, land system change, and change in biosphere integrity. These systems and processes are often called ’boundaries’ in the PB context (for further disambiguation, see Supplementary Table 1).
While all important individually, the nine PBs can be grouped into various categories. For instance, the ‘core boundaries’18 (change in biosphere integrity, climate change) control the Earth system state through geological time52; introduction of novel entities also takes on the characteristics of a core boundary during the Anthropocene19 given its potential to change Earth system function through effects on the biosphere or by altering the Earth’s energy balance53. Earth system impacts can also be separated into those that result from extraction and use of resources (freshwater change, land system change, change in biosphere integrity) versus the release and introduction of waste products (the remaining six). The PBs can also be grouped thematically. The biosphere-related boundaries (freshwater change, change in biosphere integrity, biogeochemical flows, land system change), for example, are all associated with local-to-regional-scale tipping, and might contribute to planetary-scale state shift through Earth system interactions and regulation of energy and element flows. Moreover, the climate-related boundaries (climate change, stratospheric ozone depletion, ocean acidification) are all scientifically based on evidence of their importance in regulating the state of the planet and risks of crossing climate tipping points6.
The nine PB processes are quantified by one or more control variables. These control variables are necessary to set a boundary value. Beyond this value, quantified based on the precautionary principle, there is a zone of increasing risk, wherein the lower range demarks the safe boundary and the upper range entry into the high-risk zone; these zones are based on an assessment of the range of uncertainty. Critical debates and advancing scientific knowledge have led to improved definitions and the introduction of new control variables (Table 1 and Fig. 2), particularly for freshwater change54,55,56,57,58,59, biosphere integrity60, biogeochemical flows61,62 and novel entities53.
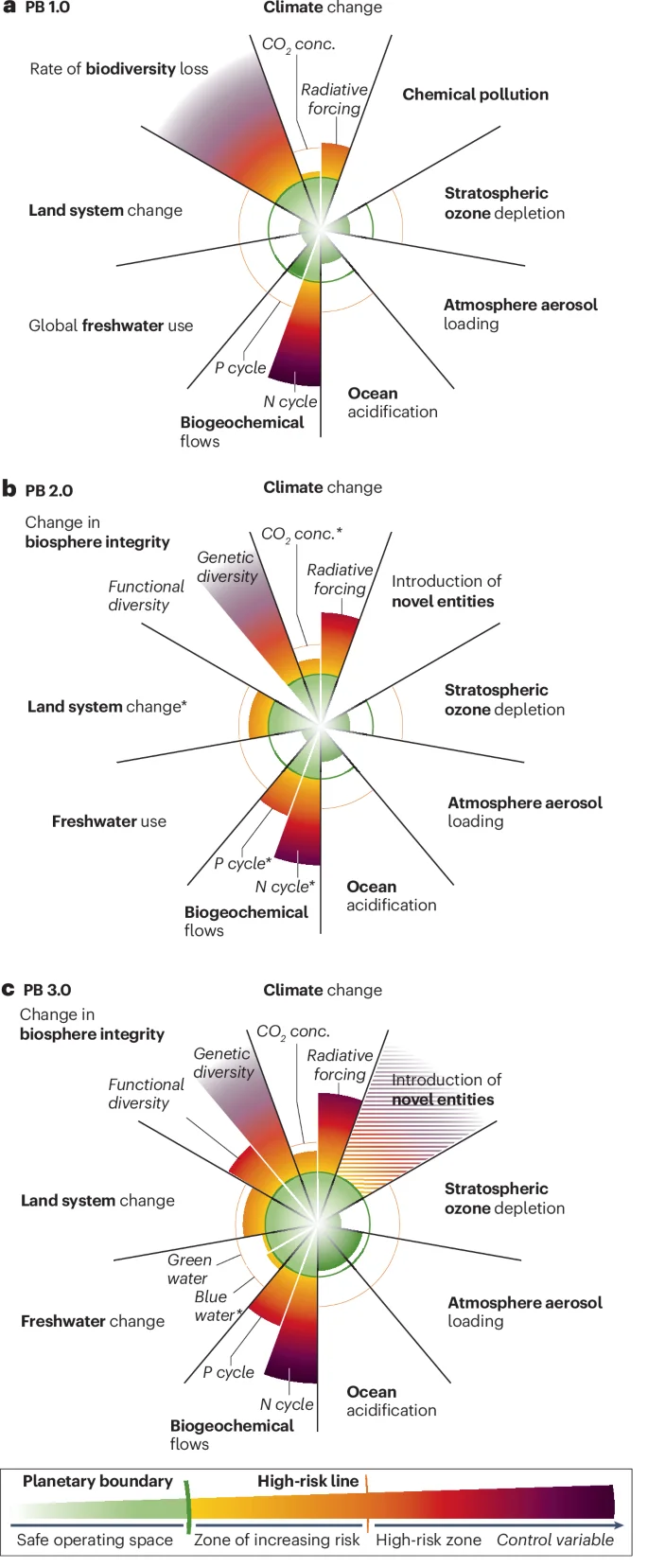
Status of the Planetary Boundaries (PBs) during PB 1.011,12 (part a), PB 2.018 (part b) and PB 3.019 (part c), as represented by the length (logarithmically scaled) and colour of the individual wedges; see also Table 1 for important details. The colour scale used is inspired by PB 3.0 but places the upper end of the zone of increasing risk (high-risk line) at dark orange. Control variables for the PB processes are normalized to the zone of increasing risk, such that their boundary values (green) and high-risk (orange) lines are consistent; this PB 2.0-type normalization contrasts to the Holocene-oriented normalization of PB 3.0. Improved understanding of Earth system interactions and feedbacks leading to updated definitions of control variables (asterisk), and updates in quantifications of the current status, all reinforce rising Earth system risks facing humanity.
The freshwater change PB, which encapsulates the global hydrological cycle, has witnessed various changes from PB 1.0 to PB 3.0 (Table 1 and Fig. 2). The PB process and the control variable were originally called ‘freshwater use’ — the globally aggregated consumptive use of blue water (rivers, lakes, reservoirs and renewable groundwater stores). In response to the need for better representation of the functions of freshwater for flow-dependent ecosystems55, the minimum percentage of mean monthly river flow was added as a control variable for PB 2.0. However, there was still a need to better represent the broader role of freshwater in supporting and regulating regional climate patterns, moisture feedback, biomass production, carbon uptake and biodiversity57,63, including the role of green water (soil moisture). For PB 3.0, the boundary was therefore renamed ‘freshwater change’, and its control variables revised to be the fractions of ice-free land areas with green or blue water changes that exit Holocene-like baseline conditions19,59,64.
Biosphere Integrity has also seen major updates (Table 1 and Fig. 2). In PB 1.0, the biosphere was incorporated into the PB framework as ‘biodiversity’, the only control variable at the time being rate of species extinctions (genetic diversity). However, genetic diversity does not capture the function of living organisms, initiating the provisional use of the Biodiversity Intactness Index65 as a second control variable for PB 2.0; the name of the PB was also changed to ‘biosphere integrity’. The Biodiversity Intactness Index was limited in that it requires expert elicitation for historical changes in species abundances, impossible for many ecosystems including the ocean, and that it does not correlate well with anthropogenic perturbation of the Earth system66. Therefore, PB 3.0 recommended human appropriation of net primary production (HANPP)67 as the control variable for functional biosphere integrity, particularly given that the capture and transport of carbon is one of the most important biosphere functions contributing to Earth system state. HANPP had also been suggested as a separate boundary for the framework68.
Control variables for biogeochemical flows — which currently captures human perturbation of the global nitrogen and phosphorus cycles — have further evolved (Table 1 and Fig. 2). For PB 1.0, it was recognized that the phosphorus control variable successfully measured the global risk for anoxic events in the ocean. However, concerns were raised that major damage would have already taken place upstream in freshwater ecosystems prior to ocean anoxia61. As a result, PB 2.0 added the regional control variable measuring phosphorus flow from fertilizers to erodible soils. Nitrogen cycle metrics were changed to include industrial and intentional biological fixation of nitrogen (Table 1). The framework keeps open the possibility of including other global element flows heavily perturbed by human activities18.
The novel entities PB has other challenges. In contrast to most PBs, the novel entities boundary cannot be established based on understanding of how the Earth system has performed during the Holocene, as these entities did not exist. Focus is therefore given to the release of synthetic chemicals as the control variable, the boundary being zero emission of synthetic chemicals that have not undergone a strenuous testing of potential ecosystem effects prior their release in the open environment53. This PB has also evolved (Table 1 and Fig. 2). Originally, the PB was named ‘chemical pollution’ (described by examples, yet still undefined), but it evolved in PB 2.0 to include the introduction of human-created chemicals and entities (such as gene-modified organisms) to the environment, and the increased mobilization of naturally occurring elements and entities. In PB 3.0, this boundary was restricted to include only anthropogenically introduced entities that are truly novel, an appropriate change given that mobilization of naturally occurring elements (for example heavy metals) could be included in the biogeochemical flows boundary19, should evidence arise of threats by them to Earth system functions.
Other PBs and their control variables have seen minor updates (Table 1). Land system change, where the control variable changed from cropland to remaining forest cover, shifted focus in PB 2.0 to biogeophysical processes in land systems that directly regulate climate. Global values are a weighted average of the three individual biome boundaries and their uncertainty zones: tropical, temperate and boreal biomes, with tropical and boreal forests identified as being more important in determining Earth system state than temperate. For aerosol loading, a global control variable could not be defined before PB 3.0. The climate change boundary (with the control variables of radiative forcing and atmospheric CO2 concentration) has seen an update for the high-risk line in PB 2.0 — it had to be brought down from 550 ppm to 450 ppm to account for new insights about risks arising from elevated greenhouse gas concentrations.
Yet other PBs have remained more static (Table 1). For instance, the stratospheric ozone (stratospheric O3 concentration) and ocean acidification (carbonate ion concentration, average global surface ocean saturation state with respect to aragonite) boundaries are little changed from PB 1.0 to PB 3.0. Further knowledge could see more changes emerge in the future, and potentially the addition of new control variables. Beyond HANPP68, additional PBs have been proposed for ocean circulation69 and soil degradation70; at present none of these proposals relates to Earth system processes that are not already captured by the framework, and they have therefore not been adopted.
The three PB assessments have made it clear that the boundaries to safe functioning of the Earth system are increasingly being transgressed. Earth observations and analytics make these assessments possible, with quantification of all nine PBs being first achieved for PB 3.0 (ref. 19). With the exception of stratospheric ozone depletion, the state of PBs has worsened over time (Table 1 and Fig. 2). Six of the nine PBs are now transgressed: climate change, introduction of novel entities, change in biogeochemical flows, freshwater change, land system change, and change in biosphere integrity. The transgressions of the boundaries of land use change, biosphere integrity and climate change are together eroding the ecological stability of large Earth-system-regulating biomes, such as the temperate, boreal and tropical forest systems. The latest assessments show that biodiversity loss, deforestation and climate forcing together place large parts of the Amazon basin at risk for crossing an irreversible tipping point towards a state of low tree cover71. Similarly, overuse of blue water and changes in green water flows, combined and interlinked with climate change, is drying out landscapes, triggering disease outbreaks in forests (for example bark beetle outbreaks) and vast forest fires63. The transgression of the global nitrogen and phosphorus boundaries causes eutrophication of freshwater lakes and rivers across the world and dead zones in marine coastal ecosystems, together eroding the ecological functions in aquatic ecosystems across the planet.
Knowledge of simultaneous transgressions of multiple PBs has put increasing attention on their resulting interactions, cascading effects and (mostly positive) feedbacks72,73,74. For example, transgressing terrestrial components of the land system change, freshwater change, changes in biogeochemical cycling and biosphere integrity PBs will reduce the tolerable climate forcing for a safe climate boundary73. Land–climate boundary interactions, in particular, are of critical importance for delineating the size and shape of the safe operating space19.
Mainstreaming PB thinking
The PBs have attracted vast interest and engagement from a diverse and widened group of scientists, becoming mainstream across disciplines addressing global sustainability challenges (Figs. 1 and 3). Although based entirely on natural science at its core, the PB framework has inspired interdisciplinary research across the social sciences and humanities (Supplementary Figs. 1 and 2), being picked up in health sciences, education, philosophy, religious studies, psychology, law, governance, behavioural science, economics and creative arts. The broad adoption of the PB framework across Earth system science, justice and governance, economics and planetary health (Fig. 3) is now discussed
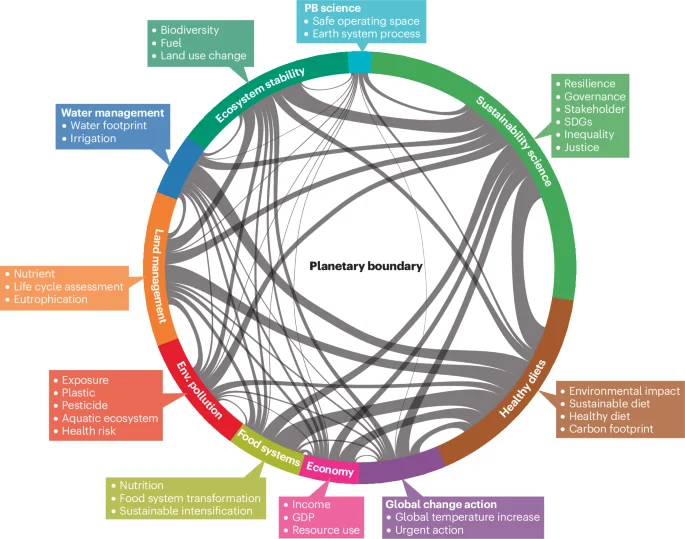
Network analysis of terms co-occurring with ‘Planetary Boundary’ in 2,500 relevant scientific publications, societal and policy reports (Supplementary methods). Terms are clustered into 10 categories (for all terms falling into these categories, see Supplementary Table 3), with the size of each category segment representing how often the terms co-occur with ‘Planetary Boundary’, and the width of the line indicating the overall strength of connections between categories. PB science has gone beyond sustainability science, influencing science and policy in health and economics. GDP, gross domestic product; SDGs, Sustainable Development Goals.
Earth system science
The PB framework has substantially contributed to the development of Earth system science. In particular, it has improved understanding of the deeply intertwined and multi-scale interactions of the physical climate system with the biosphere75,76,77,78, and importantly, identified limitations in modelling their interactions72,73,79,80. Applied modelling work has further advanced knowledge of interacting PBs to, for example, assess the potential of feeding the world population81 or creating biomass-based carbon sinks for climate change mitigation82, all within the bounds of PBs. The PBs have also highlighted knowledge gaps in certain aspects of Earth system science, specifically with regards to functioning and effects of accumulating novel entities53. For example, it remains unclear whether certain novel entities can affect ocean chemistry in a way that alters the formation of sea spray (aerosols formed from seawater at the air–sea interface), which, in turn, is an important component of the climate system.
Rising evidence of human pressures at the planetary scale — informed by the PBs — has made it clear that resilience as buffering capacity is important to preserve life-support functions. Concepts such as ‘stewardship of the Earth’s life-support systems’ and ‘stewardship of the Earth system’18,83 have thus become popular, reconnecting socioeconomic development with non-negotiable Earth system functions75. Together, human–environment or social-ecological research has ‘entered the Anthropocene’ and connects scales from local ecosystems to global PBs. An improved understanding of the planetary-scale resilience capacity provided by the biosphere, such as large-scale forest, wetland or phytoplankton ecosystems acting as carbon sinks and green water storage providing buffering capacity against human pressures, is much needed.
Earth system justice and governance
Beyond informing Earth system science, the PB framework has highlighted the need to articulate and implement Earth system justice: all people, current and future generations, have a right to live on a stable and resilient planet that remains within the safe operating space84. It comprises substantive and procedural components: substantive justice aims to ensure minimum access to natural resources (represented by the individual PBs) while reducing harm to people and allocating responsibilities fairly; procedural justice focuses on the need and right of people to have access to information, participate in decision making, and have legal and civic protection.
Achieving Earth system justice stresses the need for equity, considering minimum access rights85, affluence86 and Global North/Global South perspectives87. Setting absolute boundaries at the planetary scale translates to finite budgets for all domains, immediately raising the challenge of fair distribution of resources. This issue is prominent in the climate policy arena, determining, for example, how to compensate low-emitting countries whose fair shares of the safe carbon budget has been appropriated by nations with excess emissions88.
Ensuring ‘no significant harm’ to people from Earth system change represents another necessary condition for Earth system justice. The safe and just Earth system boundaries5 (ESBs) are an approach to combine the biophysical, planetary safety assessment from the PBs with a social science justice assessment for the same systems and processes. So far, the social science assessment has complemented five of the nine PBs (called safe ESBs in this context) with just boundaries: climate change, freshwater change, change in biosphere integrity, biogeochemical flows and aerosol loading. For the case of biosphere integrity, freshwater change and the phosphorus component of biogeochemical flows, the just ESBs are placed at the same level as the safe ESBs. Thus, substantial harm to people coincides with when these PB systems are likely to start losing their stability and function — there is strong alignment between Earth system stability and human justice. For climate change, aerosol loading and the nitrogen component of biogeochemical flows, however, the just ESBs are set at a lower, more stringent level than the safe ESBs. For example, the safe ESB for climate change is set at <1.5 °C, while the just ESB is set at 1 °C; given that global mean surface temperature rise reached 1.48 °C in 2023 (ref. 89), humanity is deep in the global climate crisis in terms of safety and justice.
The PB framework has also triggered new thinking in Earth system governance90,91. Indeed, it has been argued that a reform of international environmental governance is required90 to navigate human development in the Anthropocene, and that a framework such as the PBs can be an element of such global governance.
The quantification of a safe operating space defined by PBs has further inspired suggestions of goal-oriented international environmental law instruments as tools to strengthen multilateral agreements92, and triggered new thinking of how to strengthen environmental law at the Earth system scale93,94. An example is the integration of environmental law and PB science into the ‘Planetary Condominium’ legal framework that balances private and collective assets95.
Although often framed from the perspectives of opportunities, critiques of the PB framework for policy and governance have also emerged. These critiques relate to implications of uncertainties in Earth system science on reaching consensus in policy, and difficulties in governing PBs as they imply transferring certain sovereignty from national to a collective global level96. Also, agreeing on adopting, and thereby governing, PBs raises concerns of political conflicts97.
The Earth system science of risks in the Anthropocene, which forms the basis of the PB framework, so far outpaces the capacity of institutions and political systems to adequately balance national, regional and global governance of sustainability and planetary stability. That said, it is not as if global governance of certain PBs has not been attempted. The 1987 Montreal Protocol is a global governance regime for the stratospheric ozone boundary (established well before the PB framework was formulated); the Paris Climate Agreement is a legally binding framework for the climate boundary; and the Kunming–Montreal UN CBD Global Biodiversity Framework (from the 2022 UN Biodiversity Conference COP15) is a legal framework for the biosphere integrity boundary.
New ideas for global governance, at the interface between PBs and global governance, are closely linked to governance of the global commons (common pool resources) to be managed within finite limits. However, global commons are currently only recognized (and associated with legal frameworks) for systems beyond national jurisdictions, including Antarctica, the high seas (and deep seabed), the atmosphere and outer space. Based on the PB framework, it has been argued that the global commons ought to include all major biophysical systems on Earth that regulate its state and functioning98. Thus, the complementary planetary commons arose. The concept puts emphasis on collective PB resources that everyone, irrespective of sovereignty or jurisdiction, depends on for the stability of the Earth system99, moving away from an exploitable common pool of resources. Tipping elements, such as the Amazon rainforest and Boreal permafrost, thereby qualify as planetary global commons. With this change comes the challenge of how to govern the planetary commons, and particularly, how to ensure governance is equitable and economically fair given scarcities arising from finite PB budgets. New approaches and legal frameworks at the national and global scale will be required.
Contribution to economic thinking
The understanding of PBs as finite planetary commons calls for their integration into economic theory100 and reinforces the need for applying strong sustainability principles. The feasibility and/or necessity of strong as opposed to ‘weak’ (which assume that many natural resources can be substituted by others) sustainability measures in order to navigate in our world has been debated. Because human activities are affecting the environment at the planetary level and with respect to such a wide range of systems and processes, the PB framework provides additional scientific evidence to the longstanding debate on weak versus strong sustainability policy, in favour of the latter — at least in terms of necessity. This need implies economics that operate within PBs, which — expressed in economic terms — are finite boundaries for natural capital101,102,103.
Translating PBs to finite budgets (for carbon, nitrogen, phosphorus, land use, and use of green and blue water) creates scarcities with implications on cost and trade. Ecological economists have thus been inspired to rethink the relation between three basic economic goals of sustainable scale, just distribution and efficient allocation104. In other words, the PB framework informs welfare economics by posing cost-effectiveness and global resource limitations100,105. The finite global natural resource budgets, arising from ‘operating’ within PBs, has triggered attempts of expanding — from climate to all PBs — the assessment of investment opportunities using cost-abatement curve methods106.
The doughnut economics concept107,108 connects these finite budgets with necessary requirements for human wellbeing. It adopts the PBs as the outer ring of the ‘doughnut’ defining the ‘ceiling’ of the operating space for economies. The floor (the inner circle of the doughnut) is defined by agreed social targets such as the Sustainable Development Goals (SDGs). That approach differs substantially from the safe and just ESBs. Doughnut economics, like steady-state economics, builds on the notion of questioning infinite economic growth as it is currently understood on a finite planet. The PB framework, based on Earth system evidence and tipping point risk assessment, strengthens the call to create regenerative economies that operate within the cycles of a Holocene-like Earth-system109, resulting in finite guardrails for the economy.
The PB and doughnut economics frameworks have been further developed into the ‘good lives for all within planetary boundaries’ initiative. Here, pragmatic interpretations of the PB quantifications (using proxy control variables where data are available at country level) are compared for more than 150 countries against established indicators of ‘good human lives’ (access to food, education, equality, democratic quality, social support and so on)110,111 (Supplementary Table 2). This initiative represents a key advancement in applying concepts of human development within PBs in real economic assessments. Other academic contributions to the debate concerning alternative growth paradigms also draw on the PB framework112,113,114,115,116.
The relationship of PBs in terms of sustainability to these other concepts is straightforward: if there is a biophysical ceiling (defined by PBs) on Earth, then there must also be a social floor (defined by equity and justice).
Sustainable development research and planetary health
Increasingly, achieving SDGs within PBs has become an explicit articulation of a people–planet approach to global sustainable development (Fig. 3), addressing the need to stay within PBs to reduce inequality, limit ecological damage and secure resilience117,118. A ‘wedding cake’ version of the SDGs visualizes this articulation119. It argues that the 17 SDGs form a hierarchy: there is a PB base layer represented by the freshwater (SDG 6), climate (SDG 13), ocean (SDG 14) and biodiversity (SDG 15) goals, with social, economic and governance goals forming integrated layers that must deliver inside the non-negotiable safe space of the planetary SDG goals.
Sustainable development research, aiming to identify ways to put the abovementioned goals into practice, has to deal with a fundamental dilemma of responsibilities. Transgressions of global-scale boundaries are the result of the aggregation of widespread local-to-regional-scale environmental impacts. Thus, for the PB framework to inform governance of global and planetary commons, it must be operationalized in some format at subglobal levels120, encompassing two tasks. First, PBs must be translated into operational limits or targets, perhaps as per-capita allocations to historic responsibilities and economic capacities121; the PBs’ finite budgets of ecological space need to be shared in an equitable way, for example through accounting systems based on quota allocations for global resource use122. Second, the local-to-global chain of impacts must be addressed. The PB framework provides absolute limits of acceptable impacts for environmental management and has therefore been linked to life-cycle assessment applications. For example, it has inspired the development of methods aimed at quantifying demand for global natural resources arising from individual products and services, or individual sectors or governance units, such as nations or cities123,124. This work of applying PB framing in natural resource use has been supported by the downscaling of PBs to the national level125,126,127, connecting the two parts of the operationalization task.
The PB framework also inspired a new scientific field — planetary health. The field integrates human and planetary health, recognizing that transgressions of PBs present risks to Earth system functioning and human health. For instance, shifts in PBs present risks for heat stress, infectious diseases, food and water insecurity, pollution-related disease and mental disorders. The idea for planetary health was motivated by the Lancet Planetary Health commission report launching ‘a new science for exceptional action’128,129, and gained immediate traction. This integration of human and planetary health is exemplified by the ‘planetary health diet’, which quantifies healthy diets based on sustainable food systems, where the environmental quantifications were guided by the PB framework23. The strong scientific connections between PBs and planetary health have been further advanced in high-level health policy. For instance, the WHO Council on the Economics of Health for All used the PB framework to argue that economics needs to be rethought, putting human health at the centre by integrating planetary and human health130. Moreover, the Royal Netherlands Academy of Arts and Sciences called for prioritizing research in the emerging and increasingly broad field of planetary health for understanding and guiding actions to safeguard both human and planetary health across sectors (including energy, food, health and transport)131.
Mainstreaming PBs in society
In addition to scientific discourse, the PB framework is reflected in societal narratives of global environmental change and sustainability (Fig. 1), highlighting biophysical boundaries that socioeconomic development should not cross132,133. Indeed, PBs are increasingly embedded within global policy, national assessments and business sectors.
Owing to their science-based assessment of the global resource base, PBs have informed various global policies. In particular, the PBs feature heavily in UN frameworks or contexts, including: the UN SDGs134, UN Convention on Biological Diversity (UN CBD)135, state of the planet assessments in the UN Environment’s Global Environment Outlook136,137 (GEO 5 and 6) and UN Office for Disaster Risk Reduction (UNDRR) mid-term review of the Sendai Framework138.
The formulation of the SDGs134,139 — which provide a universal agenda integrating aspirational goals for people with sustainable global targets for the planet — was informed by the PBs through the High-Level Panel on Global Sustainability20, the Rio+20 UN Summit and the Planet Under Pressure conference (https://ccafs.cgiar.org/events/planet-under-pressure) (Fig. 1). During the work of the UN High-Level Panel on Global Sustainability20, which laid the foundation for the decision at UN Rio+20 Summit in 2012 to transition the Millennium Development Goals into SDGs, PB science had a central role. A PB science-informed working session was held at the Royal Swedish Academy of Science in 2011 (ref. 140), between the 2nd Nobel Symposium on Global Sustainability and the UN High-Level Panel. The PB 1.0 paper and the Nobel Symposium created a scientific synthesis of the necessity of integrating social and ecological resilience at the planetary scale, providing guidance to the UN High-level Panel reporting to the UN Rio+20 Summit (Resilient People, Resilient Planet; https://sustainabledevelopment.un.org/). It is likely that this interaction between PB science and global policy contributed to the integration of global sustainability targets into the human aspirations (eradicating poverty, hunger, good health, peace, and dignified socioeconomic development for all) set out in the SDGs (SDGs 6, 13, 14 and 15, on freshwater, biodiversity, oceans and climate).
The UN CBD — which aims for the conservation of biological diversity, the sustainable use of its components, and the fair and equitable sharing of benefits arising out of the use of genetic resources — is also aligned with the PBs. The UN CBD target to halt and reverse loss of biodiversity matches the genetic diversity boundary for Biosphere Integrity. Moreover, the UN CBD target to preserve 30% of intact nature on land and in the ocean translates the Land System Change boundary into a science-aligned quantitative policy target141, and at the same time, aligns with HANPP, the control variable for functional Biosphere Integrity. The PB framework also informs global policy through international initiatives and partnerships between science, businesses and other actors, as evidenced by the Global Commons Alliance (https://globalcommonsalliance.org/), the Global Commons Stewardship Framework142 and the Climate Governance Commission (https://globalgovernanceforum.org/climate-governance-commission/). In all of these initiatives, PBs form the scientific basis, guiding the focal policy areas (taking an Earth-system-wide approach to governance, business, and development) and providing quantitative ‘dashboards’ for accounting.
Several regions, nations and cities have further used the PB framework to assess their global environmental impacts or have aligned their environmental aspirations with it (Fig. 1). Uptake is particularly prominent in the European Union. Indeed, the PBs form the basis of legally binding EU action plans (the 7th and 8th European Environment Action Programme: ‘living well, within planetary boundaries’; https://environment.ec.europa.eu/strategy/environment-action-programme-2030_en), and scientific assessments of environmental footprints (‘Is Europe living within the limits of our planet?’)143. National Environmental Protection Agencies of EU countries, including Sweden144 and the Netherlands145, have further separately assessed the contribution of their respective countries to the PBs, and a similar assessment is planned for Switzerland146. Switzerland also explicitly expressed the need to respect PBs, as similarly acknowledged by the Wellbeing Economy Governments partnership (comprising Scotland, New Zealand, Iceland, Wales, Finland and Canada; https://weall.org/wego). In cities, the PB framework is primarily applied through the doughnut economics concept107,108, the tools and concepts of which are adopted in local governance (https://doughnuteconomics.org/themes/1) to align residents’ aspirations for good lives in a healthy local environment with their global responsibility to respect the wellbeing of all people within PBs111.
The PB framework similarly resonates with some segments of the business community. Indeed, the World Business Council for Sustainable Development incorporated PBs into its 2050 vision147. Various sectors are also undertaking work to internalize PB thinking, including: the food industry (Unilever, PepsiCo, DSM and Walmart), fashion (H&M, Patagonia and Houdini), beauty (L’Oréal and Kering) and technology (Ericsson and Hitachi). Moreover, many major financial consultancy firms (such as McKinsey, Arup and BCG) now profile PBs in their messaging regarding services, and a growing number are referring to the framework in their sustainability reporting.
The Science Based Targets initiative (SBTi) and Science Based Targets Network (SBTN)22 provide a concrete example of how PBs have inspired thinking about the future. The SBTi translates the finite global carbon budget into mitigation pathways (approximately 50% emission reductions by 2030 and net zero by 2050 to align with science)148, with the SBTN doing the same for PBs, translating global boundaries into operational targets for businesses and cities. As of August 2024, SBTN has, in addition to existing methods for climate, released methods for target setting for water (including nitrogen and phosphorus) and land — with targets for the ocean forthcoming. Companies and cities can apply these methods to identify their individual targets. However, the increasing uptake of quantitative PB-informed science-based targets does raise concerns over equity (fair distribution of responsibility), transparency and democratic control149.
Guiding transformation pathways
The PB framework not only informs current management, such as through science-based targets, it also serves as a guide for a ‘landing zone’ for trajectories of future development40 (Fig. 4). The framework has informed paradigm shifts on human relations to the planet, and several transformation-pathway initiatives across economic sectors, from national to regional and global scales.
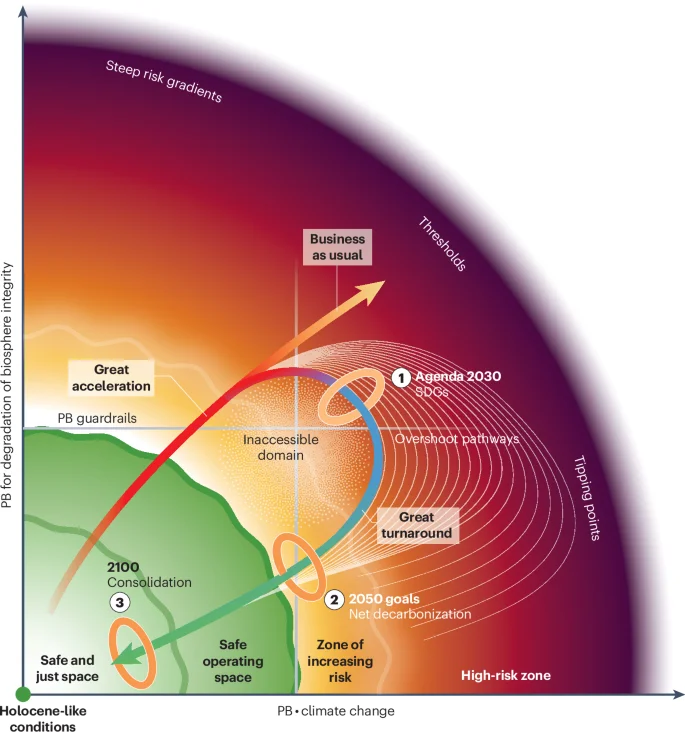
Coloured arrows represent hypothetical trajectories for the co-occurring planetary boundaries on climate change and change in biosphere integrity: the great acceleration (red arrow) has moved the Earth system from the safe operating space (green) into the zone of increasing (yellow) or high risk (red–purple). This trajectory could continue with business as usual (orange–yellow arrow) or, through political goals and targets that accelerate climate change mitigation and biosphere regeneration, exhibit the ‘great turnaround’ (blue to green arrow) whereby the Earth system returns to the safe operating space. White lines represent alternative future Earth system trajectories with increasing transgression of planetary boundaries, and orange rings important political goals and targets in the years 2030, 2050 and 2100. Planetary Boundary science highlights that to stay clear of the high-risk zone, an integrated approach to Earth system analysis and governance is needed, taking into account the complex interactions and feedbacks between climate, biosphere integrity and the collective dynamics of human societies. SDGs, Sustainable Development Goals.
The PB framework has inspired deeper thinking about needed transformations in economics100, philosophy150 and social science151. Interdisciplinary sustainability research has argued for the need to reconnect the human world with the living biosphere152, impacting on all facets of socioeconomic development. The work of the Earth Commission on quantifying justice across planetary boundaries, addressing significant harm and equitable access levels within finite global boundaries, represents social science advancements inspired by the PB framework.
The challenges of shifting social paradigms to navigate human development in a world of increasing Earth system risks have been discussed in the past within philosophical concepts and systems thinking such as the Gaia theory38 and steady-state economics101. Today, the PBs inform such philosophical discussions, for example the need to find new collective ways for humanity to inhabit Earth153. Also, the safe operating space has been presented as a target in considerations about a prevailing ‘ingenuity gap’154, which describes the inability to speed up and scale development and innovation enough to keep pace with ongoing changes to the functions of the Earth system155. Similarly, the urgent need to engage grassroots innovation also in social transitions within PBs has been emphasized156. Another example is the emerging concept of ‘provisioning systems’157, providing evidence as to why and how to transform distribution of resource allocations to satisfy social needs within PBs.
These social and philosophical efforts of aligning development discourses with a stable planet inform multiple examples of development or transformation pathways that integrate SDGs and PBs. Deep socioeconomic transformation is required to achieve the SDGs and to maintain societal development within the safe operating space158. Early examples include back-casting explorations with the Tellus Polestar model159 and explicit assessments with the IMAGE model160. Further efforts are demonstrated by The World in 2050 initiative: from a transformation-pathway assessment (guided by socioeconomic-pathway-informed sustainable development pathways)25, systematic targets emerged that translated the 169 objectives of the 17 SDGs into quantified world development and Earth system indicators161 (Fig. 4) — a guide to meet SDGs within PBs. The synergies and trade-offs between SDG and PB targets have also been explored using an SDG narrative162.
These transformation pathways can also be used to explore individual SDGs. Those related to food are particularly relevant given that the food system is the dominant cause of PB transgression163,164 and that unhealthy food is the single largest cause of premature deaths worldwide at 10–11 million deaths per year (refs. 23,165). The PBs provide a target space for transformation of the food system, setting universal boundaries and thereby advancing a planetary health diet23. To remain within PBs, nothing less than a systemic and global transformation, including the adoption of healthy diets (less animal protein, more plant-based calories), radically cutting food waste, and transitioning towards regenerative agricultural practices, is required to eradicate hunger and improve human nutrition and food security23,81,164,166,167,168,169,170,171. PB science also provides the boundary conditions for the transformation of the industrial sector towards circular and decarbonized business models that adhere to sustainable production and consumption principles172. Several initiatives158,172,173 have used PB science to set the boundaries for industrial development (across novel entities, climate change, biosphere integrity and land use change).
In terms of feasibility, a broader picture has been painted by a 50-year update of the Limits to Growth report32, and the Earth for All initiative explored transformation pathways that foster world development within PBs173. Five ‘turnarounds’ across energy, food, equity, finance and empowerment were quantitatively assessed against PBs. Even with very optimistic projections of sustainable transitions that deliver equitable economic development and achieve the SDGs, a return to the safe operating space is barely within reach, in particular for climate and biosphere integrity173. These challenges are also reflected in the emerging focus of seeking positive social tipping points that accelerate transformation pathways back into the safe operating space174,175.
Summary and future perspectives
The PB framework identifies, defines, quantifies and offers pathways toward the safe operating space for humanity — with stable, quasi-equilibrium conditions not vastly different from those experienced during the Holocene. PBs have become integral to the way global sustainability is understood and addressed, influencing research across the social and natural sciences, and having applications in policy, business and civil society. Despite its widespread adoption, the PB framework will continue to evolve as new knowledge about the Earth system and human–Earth-system interactions becomes available.
Many scientific challenges remain with respect to advancing the PB framework, including the need for improved quantifications. For instance, the demarcation of the upper edge of the zone of increasing risk (called the high-risk line) is currently associated with more uncertainty than the placement of the safe PB for many variables. Also, the zone of increasing risk (between the PB and the high-risk line) is characterized by uncertainty about the exact degree of risk (Supplementary Table 1). For genetic diversity, the safe boundary and high-risk line are 10 and 100 extinctions per million species-years, respectively, and for climate forcing, 1 and 1.5 W m−2, respectively. Nevertheless, there is high confidence that biophysical boundaries at the Earth system scale do exist. Still, this uncertainty needs to be reduced, by advancing scientific analyses of Earth resilience, and in particular Earth system feedbacks between PB processes. A Tipping Point Model Intercomparison Project (TIPMIP) has been launched to increase the understanding of how Earth system interactions affect the risks of crossing planet-regulating tipping points, which, in turn, will help to reduce the uncertainty range in setting PBs. Earth system modelling on PB interactions is also of critical importance in verifying and improving quantifications of the safe operating space.
More explicit quantifications of PBs with respect to the Holocene are also necessary. PB science is premised on the notion that the Holocene is the only state known for certain to support the world as it is known and is, thus, the reference state for setting PBs. Yet only the safe boundary for the climate change PB has been explicitly assessed against the Holocene. Other boundary processes are increasingly adopting this approach, including defining control variables for green and blue water for the freshwater change boundary59,64 and quantifying the safe level for functional diversity for biosphere integrity5,19. PB science should continue to move towards a generic method development across all PBs, where maximum allowed deviation from the Holocene range of variability becomes a guiding principle. Doing so is a major scientific challenge given the lack of data on Holocene range of variability across all biosphere PBs, but improved Earth system modelling and closer collaboration between Earth system science and palaeosciences can shed important light on the ‘corridor of life’ for all PBs during the Holocene.
Moreover, the interaction between PBs and the concurrent transgression of multiple PBs needs improved assessment. At present, the PBs are often assessed in isolation, with the implications of simultaneous transgression of multiple boundaries little examined19,73. In particular, there is a need to assess the impacts of lost biosphere resilience (responding to change in biosphere integrity, land system change, ocean acidification, freshwater change, introduction of novel entities, and change in biogeochemical flows) on the climate boundary, and how these biosphere-induced climate stressors might cause feedbacks that, in turn, further undermine biosphere resilience. Coupled Earth system models are required to assess how various interactions influence each other, and to identify key entry points to cut short harmful causal chains.
The aforementioned aspects would all benefit from high-resolution Earth observation being better connected to the PBs. Indeed, linking Earth observation analytics could potentially provide global assessments of transgressions in aggregated form, as well as spatially distributed high-resolution maps. Furthermore, connecting with Earth observations would allow opportunities for new control variables to be added for each PB, as done with the subdivision of blue and green water for freshwater change. These control variables must be relevant indicators for safety at the planetary scale, while also having the potential to be scaled to, and thereby implemented at, national, sectoral or even the city and industry level. They should capture the function of ocean biology and land use activities (beyond deforestation), and in all cases must be carefully evaluated to avoid cherry-picking and ensure their ability to support the intention of the PB framework. These developments would not only contribute to improving the precision and validation of PB risk assessments, but also increase the usefulness of the framework across scales and in different sectors (as different stakeholders use different benchmarks). Multiple control variables could further aid management and equitable sharing of responsibility for addressing pressures on spatially heterogeneous PB processes (such as land system change, freshwater change and biosphere integrity)176.
In addition to integration with Earth observations, PB-based world–Earth modelling could also help to advance the PB framework, aiming at a deeper integration of processes, interactions and feedbacks between the biophysical Earth system and human societies. ‘World’ refers here to complex human societies as embedded in the biophysical Earth system (Supplementary Table 1). Some attempts have been made in this regard173,177,178, which highlighted the need to deepen understanding of human–environment interactions at the global scale, coupling Earth system assessments with research on human agency, innovation and transformation. Social tipping points research falls in this category174. Increasingly, world–Earth modelling can be validated with new Big-Data and artificial intelligence approaches that integrate PB-wide Earth observations, with statistical neural network methodologies to test safe boundary setting and integrate human dynamics179,180.
Collectively, these actions could also contribute toward reduced time between PB updates. On average, these updates have occurred every 6–8 years, which, given the rapidly approaching tipping points, is much too long. More regular, potentially even annual, updates would provide a measurement dashboard for the planet that stakeholders can use to monitor progress towards sustainable development.
Regardless of the interval between updates, it is clear that some PBs have been transgressed and that others are increasingly at risk of being transgressed. So far, however, negative Earth-system feedbacks are working against an irreversible transition to a new state; dampening feedbacks are dominating, as evidenced by 93% of anthropogenic heat being taken up by the ocean and 56% of anthropogenic carbon being taken up by the biosphere9 (although there are signs that biosphere resilience is being lost with Brazilian part of the Amazon tipping from carbon sink to source181). It is unclear how long the Earth system can continue to buffer anthropogenic stress with multiple transgressed PBs and whether it will instead self-amplify them182. The overall resilience of the Earth system must therefore be investigated, as with the challenge to reduce uncertainties, mentioned previously. Tipping point research is the most prominent example to achieve that.
Welfare economics, sustainable development and scenario analyses of transformation pathways need to be broadened to ensure that ongoing world development occurs within safe (and just) boundaries. Currently, scenario analyses of future trajectories of world development tend to focus on single domains, such as climate and the energy transition, or land–water-biodiversity and the food transition. Yet transformation pathways that meet the ‘landing zone’ of desired outcomes for world development (SDGs) within PBs need to be explored. This broadening has started across several initiatives, pioneered by The World in 205025. A global modelling intercomparison on transformation pathways that meet the SDGs within PBs (include safe PBs and justice-based Earth system boundaries) is being undertaken under the auspices of the Earth Commission, where a wider community of global modelling teams are invited to explore transformation pathways that can meet world development within planetary boundaries5,161.
Governing and monitoring Earth’s stability and resilience is not optional, it is a necessity given the risks of planetary state being destabilized. The PB framework offers a much-needed complement to how sustainable development is understood and pursued, as evidenced by the broad scientific uptake of PB science. We add the quest to reach human prosperity and equity on a stable and resilient planet, within planetary boundaries, that provides all people, generations, communities and societies with a safe operating space on Earth.
References
-
Vitousek, P. M., Mooney, H. A., Lubchenco, J. & Melillo, J. M. Human domination of Earth’s ecosystems. Science 277, 494–499 (1997).
Google Scholar
-
Crutzen, P. J. Geology of mankind. Nature 415, 23–23 (2002).
Google Scholar
-
Steffen, W. et al. Global Change and the Earth System (Springer, 2004).
-
Steffen, W., Crutzen, P. J. & McNeill, J. R. The Anthropocene: are humans now overwhelming the great forces of nature? AMBIO J. Hum. Environ. 36, 614–621 (2007).
Google Scholar
-
Rockström, J. et al. Safe and just Earth system boundaries. Nature 619, 102–111 (2023).
Google Scholar
-
Armstrong McKay, D. I. et al. Exceeding 1.5 °C global warming could trigger multiple climate tipping points. Science 377, eabn7950 (2022).
Google Scholar
-
Scheffer, M. et al. Early-warning signals for critical transitions. Nature 461, 53–59 (2009).
Google Scholar
-
Lenton, T. M. et al. Tipping elements in the Earth’s climate system. Proc. Natl Acad. Sci. USA 105, 1786–1793 (2008).
Google Scholar
-
IPCC. Climate Change 2023: Synthesis Report (eds Lee, H. & Romero, J.) (IPCC, 2023).
-
Pörtner, H. O. et al. IPBES-IPCC Co-Sponsored Workshop Report on Biodiversity and Climate Change https://doi.org/10.5281/zenodo.4782538 (IPBES and IPCC, 2021).
-
Rockström, J. et al. A safe operating space for humanity. Nature 461, 472–475 (2009).
Google Scholar
-
Rockström, J. et al. Planetary boundaries: exploring the safe operating space for humanity. Ecol. Soc. 14, 32 (2009).
Google Scholar
-
Steffen, W. et al. Trajectories of the Earth system in the Anthropocene. Proc. Natl Acad. Sci. USA 115, 8252–8259 (2018).
Google Scholar
-
Westerhold, T. et al. An astronomically dated record of Earth’s climate and its predictability over the last 66 million years. Science 369, 1383–1387 (2020).
Google Scholar
-
Osman, M. B. et al. Globally resolved surface temperatures since the Last Glacial Maximum. Nature 599, 239–244 (2021).
Google Scholar
-
Feynman, J. & Ruzmaikin, A. Climate stability and the development of agricultural societies. Clim. Change 84, 295–311 (2007).
Google Scholar
-
Rockström, J. & Klum, M. Big World, Small Planet: Abundance within Planetary Boundaries (Yale Univ. Press, 2015).
-
Steffen, W. et al. Planetary boundaries: guiding human development on a changing planet. Science 347, 1259855 (2015).
Google Scholar
-
Richardson, K. et al. Earth beyond six of nine planetary boundaries. Sci. Adv. 9, eadh2458 (2023).
Google Scholar
-
Resilient People, Resilient Planet: A Future Worth Choosing, the Report of the United Nations Secretary-General’s High Level Panel on Global Sustainability (United Nations, 2012).
-
Living Planet Report 2016. Risk and Resilience in a New Era https://www.worldwildlife.org/pages/living-planet-report-2016 (WWF, 2016).
-
Science Based Targets Network https://sciencebasedtargetsnetwork.org/ (SBTN, 2020).
-
Willett, W. et al. Food in the Anthropocene: the EAT–Lancet Commission on healthy diets from sustainable food systems. Lancet 393, 447–492 (2019).
Google Scholar
-
Singh Chawla, D. Revealed: the ten research papers that policy documents cite most. Nature https://doi.org/10.1038/d41586-024-00660-1 (2024).
Google Scholar
-
The World in 2050 Initiative. Transformations to Achieve the Sustainable Development Goals www.twi2050.org (IIASA, 2018).
-
Earth’s boundaries? Nature 461, 447–448 (2009).
-
Turner, B. L. et al. The Earth as Transformed by Human Action — Global and Regional Changes in the Biosphere over the Past 300 Years (Cambridge Univ. Press, 1993).
-
Witze, A. Geologists reject the Anthropocene as Earth’s new epoch — after 15 years of debate. Nature 627, 249–250 (2024).
Google Scholar
-
Daly, H. E. On economics as a life science. J. Polit. Econ. 76, 392–406 (1968).
Google Scholar
-
The Cocoyoc declaration. Intl Organ. 29, 893–901 (1975).
-
Boulding, Kenneth E. in Environmental Quality in a Growing Economy 3–14 (Johns Hopkins Univ. Press, 1966); http://arachnid.biosci.utexas.edu/courses/thoc/readings/boulding_spaceshipearth.pdf.
-
Meadows, D., Meadows, D. L., Randers, J. & Behrens III, W. W. The Limits to Growth (Universe, 1972).
-
Daly, H. E. (ed.) Towards a Steady-State Economy (Freeman, 1973).
-
Steffen, W. et al. The emergence and evolution of Earth system science. Nat. Rev. Earth Environ. 1, 54–63 (2020).
Google Scholar
-
Petit, R. J. et al. Climate and atmospheric history of the past 420,000 years from the Vostok ice core, Antarctica. Nature 399, 429–413 (1999).
Google Scholar
-
Holling, C. S. Resilience and stability of ecological systems. Annu. Rev. Ecol. Syst. 4, 1–23 (1973).
Google Scholar
-
Folke, C. et al. Regime shifts, resilience, and biodiversity in ecosystem management. Annu. Rev. Ecol. Evol. Syst. 35, 557–581 (2004).
Google Scholar
-
Lovelock, J. E. Gaia as seen through the atmosphere. Atmos. Environ. 1967 6, 579–580 (1972).
-
Earth System Science — Overview: A Program for Global Change (National Academies Press, 1986).
-
Schellnhuber, H. J. ‘Earth system’ analysis and the second Copernican revolution. Nature 402, C19–C23 (1999).
Google Scholar
-
Scheffer, M., Carpenter, S., Foley, J. A., Folke, C. & Walker, B. Catastrophic shifts in ecosystems. Nature 413, 591–596 (2001).
Google Scholar
-
Downing, A. S. et al. Matching scope, purpose and uses of planetary boundaries science. Environ. Res. Lett. 14, 073005 (2019).
Google Scholar
-
Downing, A. S. et al. Learning from generations of sustainability concepts. Environ. Res. Lett. 15, 083002 (2020).
Google Scholar
-
Galli, A. et al. Questioning the ecological footprint. Ecol. Indic. 69, 224–232 (2016).
Google Scholar
-
Petschel-Held, G., Schellnhuber, H.-J., Bruckner, T., Tóth, F. L. & Hasselmann, K. The tolerable windows approach: theoretical and methodological foundations. Clim. Change 41, 303–331 (1999).
Google Scholar
-
Past Interglacials Working Group of PAGES Interglacials of the last 800,000 years. Rev. Geophys. 54, 162–219 (2016).
Google Scholar
-
Hays, J. D., Imbrie, J. & Shackleton, N. J. Variations in the Earth’s orbit: pacemaker of the ice ages. Science 194, 1121–1132 (1976).
Google Scholar
-
Dansgaard, W. et al. Evidence for general instability of past climate from a 250-kyr ice-core record. Nature 364, 218–220 (1993).
Google Scholar
-
Ruddiman, W. F. The anthropogenic greenhouse era began thousands of years ago. Clim. Change 61, 261–293 (2003).
Google Scholar
-
Oppenheimer, S. Out of Eden: The Peopling of the World (Little, Brown, 2004).
-
IPCC Climate Change 2021: The Physical Science Basis (eds Masson-Delmotte, V. et al.) (IPCC, 2021).
-
Richardson, K. & Rosing, M. in Multiplicity of Time Scales in Complex Systems: Challenges for Sciences and Communication, 215–233 (Springer Nature, 2023).
-
Persson, L. et al. Outside the safe operating space of the planetary boundary for novel entities. Environ. Sci. Technol. 56, 1510–1521 (2022).
Google Scholar
-
Molden, D. et al. Improving agricultural water productivity: between optimism and caution. Agric. Water Manag. 97, 528–535 (2010).
Google Scholar
-
Gerten, D. et al. Towards a revised planetary boundary for consumptive freshwater use: role of environmental flow requirements. Curr. Opin. Environ. Sustain. 5, 551–558 (2013).
Google Scholar
-
Bogardi, J. J., Fekete, B. M. & Vörösmarty, C. J. Planetary boundaries revisited: a view through the ‘water lens’. Curr. Opin. Environ. Sustain. 5, 581–589 (2013).
Google Scholar
-
Gleeson, T. et al. The water planetary boundary: interrogation and revision. One Earth 2, 223–234 (2020).
Google Scholar
-
Pastor, A. V. et al. Understanding the transgression of global and regional freshwater planetary boundaries. Phil. Trans. R. Soc. A 380, 20210294 (2022).
Google Scholar
-
Wang-Erlandsson, L. et al. A planetary boundary for green water. Nat. Rev. Earth Environ. 3, 380–392 (2022).
Google Scholar
-
Mace, G. M. et al. Approaches to defining a planetary boundary for biodiversity. Glob. Environ. Change 28, 289–297 (2014).
Google Scholar
-
Carpenter, S. R. & Bennett, E. M. Reconsideration of the planetary boundary for phosphorus. Environ. Res. Lett. 6, 014009 (2011).
Google Scholar
-
de Vries, W., Kros, J., Kroeze, C. & Seitzinger, S. P. Assessing planetary and regional nitrogen boundaries related to food security and adverse environmental impacts. Curr. Opin. Environ. Sustain. 5, 392–402 (2013).
Google Scholar
-
Gleeson, T. et al. Illuminating water cycle modifications and Earth system resilience in the Anthropocene. Water Resour. Res. 56, e2019WR024957 (2020).
Google Scholar
-
Porkka, M. et al. Notable shifts beyond pre-industrial streamflow and soil moisture conditions transgress the planetary boundary for freshwater change. Nat. Water 2, 262–273 (2024).
Google Scholar
-
Scholes, R. J. & Biggs, R. A biodiversity intactness index. Nature 434, 45–49 (2005).
Google Scholar
-
Martin, P. A., Green, R. E. & Balmford, A. The biodiversity intactness index may underestimate losses. Nat. Ecol. Evol. 3, 862–863 (2019).
Google Scholar
-
Haberl, H., Erb, K.-H. & Krausmann, F. Human appropriation of net primary production: patterns, trends, and planetary boundaries. Annu. Rev. Environ. Resour. 39, 363–391 (2014).
Google Scholar
-
Running, S. W. A measurable planetary boundary for the biosphere. Science 337, 1458–1459 (2012).
Google Scholar
-
Nash, K. L. et al. Planetary boundaries for a blue planet. Nat. Ecol. Evol. 1, 1625–1634 (2017).
Google Scholar
-
Kraamwinkel, C. T., Beaulieu, A., Dias, T. & Howison, R. A. Planetary limits to soil degradation. Commun. Earth Environ. 2, 249 (2021).
Google Scholar
-
Flores, B. M. et al. Critical transitions in the Amazon forest system. Nature 626, 555–564 (2024).
Google Scholar
-
Lade, S. J. et al. Potential feedbacks between loss of biosphere integrity and climate change. Glob. Sustain. 2, e21 (2019).
Google Scholar
-
Lade, S. J. et al. Human impacts on planetary boundaries amplified by Earth system interactions. Nat. Sustain. 3, 119–128 (2020).
Google Scholar
-
Anderies, J. M., Carpenter, S. R., Steffen, W. & Rockström, J. The topology of non-linear global carbon dynamics: from tipping points to planetary boundaries. Environ. Res. Lett. 8, 044048 (2013).
Google Scholar
-
Folke, C. et al. Reconnecting to the biosphere. Ambio 40, 719–738 (2011).
Google Scholar
-
Donges, J. F. et al. Closing the loop: reconnecting human dynamics to Earth system science. Anthr. Rev. 4, 151–157 (2017).
-
Earth System Science Discovery, Diagnosis, and Solutions in Times of Global Change (Nationale Akademie der Wissenschaften Leopoldina, 2022).
-
Roberts, P. et al. Mapping our reliance on the tropics can reveal the roots of the Anthropocene. Nat. Ecol. Evol. 7, 632–636 (2023).
Google Scholar
-
Purves, D. et al. Time to model all life on Earth. Nature 493, 295–297 (2013).
Google Scholar
-
Heck, V., Donges, J. F. & Lucht, W. Collateral transgression of planetary boundaries due to climate engineering by terrestrial carbon dioxide removal. Earth Syst. Dyn. 7, 783–796 (2016).
Google Scholar
-
Gerten, D. et al. Feeding ten billion people is possible within four terrestrial planetary boundaries. Nat. Sustain. 3, 200–208 (2020).
Google Scholar
-
Heck, V., Gerten, D., Lucht, W. & Popp, A. Biomass-based negative emissions difficult to reconcile with planetary boundaries. Nat. Clim. Change 8, 151–155 (2018).
Google Scholar
-
Chapin, F. S. et al. Earth stewardship: shaping a sustainable future through interacting policy and norm shifts. Ambio 51, 1907–1920 (2022).
Google Scholar
-
Gupta, J. et al. Earth system justice needed to identify and live within Earth system boundaries. Nat. Sustain. 6, 630–638 (2023).
Google Scholar
-
Rammelt, C. F. et al. Impacts of meeting minimum access on critical Earth systems amidst the Great Inequality. Nat. Sustain. 6, 212–221 (2023).
Google Scholar
-
Wiedmann, T., Lenzen, M., Keyßer, L. T. & Steinberger, J. K. Scientists’ warning on affluence. Nat. Commun. 11, 3107 (2020).
Google Scholar
-
Sultana, F. Whose growth in whose planetary boundaries? Decolonising planetary justice in the Anthropocene. Geo Geogr. Environ. 10, e00128 (2023).
Google Scholar
-
Fanning, A. L. & Hickel, J. Compensation for atmospheric appropriation. Nat. Sustain. 6, 1077–1086 (2023).
Google Scholar
-
2023 was the hottest year on record, Copernicus data show. ECMWF https://www.ecmwf.int/en/about/media-centre/news/2024/2023-was-hottest-year-record-copernicus-data-show (2024).
-
Galaz, V., Biermann, F., Folke, C., Nilsson, M. & Olsson, P. Global environmental governance and planetary boundaries: an introduction. Ecol. Econ. 81, 1–3 (2012).
Google Scholar
-
Galaz, V. Global Environmental Governance, Technology and Politics: The Anthropocene Gap (Edward Elgar, 2014).
-
Kim, R. E. & Bosselmann, K. International environmental law in the Anthropocene: towards a purposive system of multilateral environmental agreements. Transnatl. Environ. Law 2, 285–309 (2013).
Google Scholar
-
du Toit, L. & Kotzé, L. J. Reimagining international environmental law for the Anthropocene: an Earth system law perspective. Earth Syst. Gov. 11, 100132 (2022).
Google Scholar
-
French, D. & Kotzé, L. J. (eds) Research Handbook on Law, Governance and Planetary Boundaries (Edward Elgar, 2021).
-
Magalhães, P. et al. Planetary Condominium: The Legal Framework for the Common Home of Humanity (Global Challenges Foundation, 2018).
-
Biermann, F. & Kim, R. E. The boundaries of the planetary boundary framework: a critical appraisal of approaches to define a ‘safe operating space’ for humanity. Annu. Rev. Env. Resour. 45, 497–512 (2020).
Google Scholar
-
Biermann, F. et al. Navigating the Anthropocene: improving Earth system governance. Science 335, 1306–1307 (2012).
Google Scholar
-
Nakicenovic, N., Rockström, J., Gaffney, O., Zimm, C. & Kabat, P. Global Commons in the Anthropocene: World Development on a Stable and Resilient Planet. IIASA Working Paper WP-16-019 (2016).
-
Rockström, J. et al. The planetary commons: a new paradigm for safeguarding Earth-regulating systems in the Anthropocene. Proc. Natl Acad. Sci. USA 121, e2301531121 (2024).
Google Scholar
-
Sureth, M., Kalkuhl, M., Edenhofer, O. & Rockström, J. A welfare economic approach to planetary boundaries. Jb. Natl. Stat. https://doi.org/10.1515/jbnst-2022-0022 (2023).
-
Daly, H. E. Steady-State Economics (Island, 1991).
-
Turner, R. K., Perrings, C. & Folke, C. Ecological Economics: Paradigm or Perspective. CSERGE Working Paper (1995).
-
Dasgupta, P. The Economics of Biodiversity: The Dasgupta Review: Full Report (HM Treasury, 2021).
-
Daly, H. E. Allocation, distribution, and scale: towards an economics that is efficient, just, and sustainable. Ecol. Econ. 6, 185–193 (1992).
Google Scholar
-
Sterner, T. et al. Policy design for the Anthropocene. Nat. Sustain. 2, 14–21 (2019).
Google Scholar
-
McKinsey & Company. Nature in the Balance: What Companies Can Do to Restore Natural Capital (2022).
-
Raworth, K. A Safe and Just Space for Humanity: Can We Live within the Doughnut? (Oxfam, 2012).
-
Raworth, K. A doughnut for the Anthropocene: humanity’s compass in the 21st century. Lancet Planet. Health 1, e48–e49 (2017).
Google Scholar
-
Raworth, K. Doughnut Economics: Seven Ways to Think Like a 21st-Century Economist (Penguin, 2022).
-
O’Neill, D. W., Fanning, A. L., Lamb, W. F. & Steinberger, J. K. A good life for all within planetary boundaries. Nat. Sustain. 1, 88–95 (2018).
Google Scholar
-
Fanning, A. L., O’Neill, D. W., Hickel, J. & Roux, N. The social shortfall and ecological overshoot of nations. Nat. Sustain. 5, 26–36 (2022).
Google Scholar
-
van den Bergh, J. C. J. M. & Kallis, G. Growth, a-growth or degrowth to stay within planetary boundaries? J. Econ. Issues 46, 909–920 (2012).
Google Scholar
-
Haberl, H. et al. A systematic review of the evidence on decoupling of GDP, resource use and GHG emissions, part II: synthesizing the insights. Environ. Res. Lett. 15, 065003 (2020).
Google Scholar
-
Hickel, J. & Kallis, G. Is green growth possible? N. Polit. Econ. 25, 469–486 (2020).
Google Scholar
-
Hubacek, K., Chen, X., Feng, K., Wiedmann, T. & Shan, Y. Evidence of decoupling consumption-based CO2 emissions from economic growth. Adv. Appl. Energy 4, 100074 (2021).
Google Scholar
-
Jackson, T. & Victor, P. A. Unraveling the claims for (and against) green growth. Science 366, 950–951 (2019).
Google Scholar
-
Stafford-Smith, M. et al. Integration: the key to implementing the Sustainable Development Goals. Sustain. Sci. 12, 911–919 (2017).
Google Scholar
-
Bowen, K. J. et al. Implementing the ‘Sustainable Development Goals’: towards addressing three key governance challenges — collective action, trade-offs, and accountability. Curr. Opin. Environ. Sustain. 26–27, 90–96 (2017).
-
Folke, C., Biggs, R., Norström, A. V., Reyers, B. & Rockström, J. Social-ecological resilience and biosphere-based sustainability science. Ecol. Soc. 21, art41 (2016).
Google Scholar
-
Keppner, B. & Hoff, H. Planetary Boundaries: Challenges for Science, Civil Society and Politics. https://www.umweltbundesamt.de/en/publikationen/planetary-boundaries-challenges-for-science-civil (Umweltbundesamt, 2020).
-
Bai, X. et al. How to stop cities and companies causing planetary harm. Nature 609, 463–466 (2022).
Google Scholar
-
Meyer, K. & Newman, P. The Planetary Accounting Framework: a novel, quota-based approach to understanding the impacts of any scale of human activity in the context of the planetary boundaries. Sustain. Earth 1, 4 (2018).
Google Scholar
-
Ryberg, M. W., Owsianiak, M., Richardson, K. & Hauschild, M. Z. Development of a life-cycle impact assessment methodology linked to the planetary boundaries framework. Ecol. Indic. 88, 250–262 (2018).
Google Scholar
-
Hellweg, S., Benetto, E., Huijbregts, M. A. J., Verones, F. & Wood, R. Life-cycle assessment to guide solutions for the triple planetary crisis. Nat. Rev. Earth Environ. 4, 471–486 (2023).
Google Scholar
-
Parsonsová, A. Downscaling planetary boundaries to the national level: a review of methods and indicators. J. Landsc. Ecol. 14, 39–45 (2021).
Google Scholar
-
Häyhä, T., Lucas, P. L., van Vuuren, D. P., Cornell, S. E. & Hoff, H. From planetary boundaries to national fair shares of the global safe operating space — How can the scales be bridged? Glob. Environ. Change 40, 60–72 (2016).
Google Scholar
-
Hachaichi, M. & Baouni, T. Downscaling the planetary boundaries (PBs) framework to city scale-level: de-risking MENA region’s environment future. Environ. Sustain. Indic. 5, 100023 (2020).
-
Horton, R. & Lo, S. Planetary health: a new science for exceptional action. Lancet 386, 1921–1922 (2015).
Google Scholar
-
Whitmee, S. et al. Safeguarding human health in the Anthropocene epoch: report of the Rockefeller Foundation–Lancet Commission on planetary health. Lancet 386, 1973–2028 (2015).
Google Scholar
-
WHO Council on the Economics of Health for All. Health for All — Transforming Economies to Deliver What Matters: Final Report of the WHO Council on the Economics of Health for All (WHO, 2023).
-
Planetary Health. An Emerging Field to Be Developed (Royal Netherlands Academy of Arts and Sciences, 2023).
-
Brand, U. et al. From planetary to societal boundaries: an argument for collectively defined self-limitation. Sustain. Sci. Pract. Policy 17, 264–291 (2021).
-
Blühdorn, I. Planetary boundaries, societal boundaries, and collective self-limitation: moving beyond the post-Marxist comfort zone. Sustain. Sci. Pract. Policy 18, 576–589 (2022).
-
UN DESA. Transforming Our World: The 2030 Agenda for Sustainable Development. https://wedocs.unep.org/20.500.11822/11125 (UNEP, 2016).
-
Obura, D. O. et al. Achieving a nature- and people-positive future. One Earth 6, 105–117 (2023).
Google Scholar
-
UNEP. Global Environment Outlook 5 (GEO 5): Environment for the Future We Want. https://wedocs.unep.org/20.500.11822/8021 (UNEP, 2012).
-
UNEP. Global Environment Outlook 6 (GEO 6): Healthy Planet, Healthy People. https://wedocs.unep.org/20.500.11822/27539 (UNEP, 2019).
-
UNDRR. Thematic Study: Planetary Boundaries. http://sendaiframework-mtr.undrr.org/quick/76149 (UNDRR, 2022).
-
UN DESA. The Sustainable Development Goals Report 2018. https://unstats.un.org/sdgs/report/2018/ (UN, 2018).
-
Folke, C. & Rockström, J. 3rd Nobel Laureate symposium on global sustainability: transforming the world in an era of global change. AMBIO 40, 717–718 (2011).
Google Scholar
-
Kunming–Montreal Global Biodiversity Framework: Convention on Biological Diversity (2022).
-
IFI. Safeguarding the Global Commons for Human Prosperity and Environmental Sustainability. https://policycommons.net/artifacts/2455478/safeguarding-the-global-commons-for-human-prosperity-and-environmental-sustainability/3477275/ (2022).
-
EAA, Wugt Larsen, F. & Lung, T. Is Europe Living within the Limits of Our Planet? An Assessment of Europe’s Environmental Footprints in Relation to Planetary Boundaries. https://doi.org/10.2800/890673 (Publications Office of the EU, 2020).
-
Living within the Limits of Our Planet — A Swedish Perspective. https://www.naturvardsverket.se/publikationer/7000/978-91-620-7092-2/ (The Swedish Environmental Protection Agency, 2023).
-
Lucas, P. & Wilting, H. Towards a Safe Operating Space for the Netherlands: Using Planetary Boundaries to Support National Implementation of Environment-Related SDGs (PBL Netherlands Environmental Assessment Agency, 2019).
-
Dao, H., Peduzzi, P. & Friot, D. National environmental limits and footprints based on the Planetary Boundaries framework: the case of Switzerland. Glob. Environ. Change 52, 49–57 (2018).
Google Scholar
-
Vision 2050: Time to Transform. https://www.wbcsd.org/contentwbc/download/11765/177145/1 (WBCSD, 2021).
-
Watson, R. Pathways to Net-Zero — SBTi Technical Summary Version 1.0 (SBTi, 2021).
-
Tilsted, J. P., Palm, E., Bjørn, A. & Lund, J. F. Corporate climate futures in the making: why we need research on the politics of science-based targets. Energy Res. Soc. Sci. 103, 103229 (2023).
Google Scholar
-
Latour, B. & Lenton, T. M. Extending the domain of freedom, or why Gaia is so hard to understand. Crit. Inq. 45, 659–680 (2019).
Google Scholar
-
Olsson, P. & Moore, M.-L. in Positive Tipping Points Towards Sustainability: Understanding the Conditions and Strategies for Fast Decarbonization in Regions (eds Tàbara, J. D. et al.) 59–77 (Springer, 2024).
-
Folke, C. & Gunderson, L. Reconnecting to the biosphere: a social-ecological renaissance. Ecol. Soc. https://doi.org/10.5751/ES-05517-170455 (2012).
-
Latour, B. Down to Earth: Politics in the New Climatic Regime (Polity, 2018).
-
Westley, F. et al. Tipping toward sustainability: emerging pathways of transformation. Ambio 40, 762–780 (2011).
Google Scholar
-
Steffen, W., Broadgate, W., Deutsch, L., Gaffney, O. & Ludwig, C. The trajectory of the Anthropocene: the great acceleration. Anthr. Rev. 2, 81–98 (2015).
-
Leach, M. et al. Transforming innovation for sustainability. Ecol. Soc. https://doi.org/10.5751/ES-04933-170211 (2012).
-
Fanning, A. L., O’Neill, D. W. & Büchs, M. Provisioning systems for a good life within planetary boundaries. Glob. Environ. Change 64, 102135 (2020).
Google Scholar
-
Sachs, J. D. et al. Six transformations to achieve the sustainable development goals. Nat. Sustain. 2, 805–814 (2019).
Google Scholar
-
Gerst, M. D., Raskin, P. D. & Rockström, J. Contours of a resilient global future. Sustainability 6, 123–135 (2013).
Google Scholar
-
van Vuuren, D. P. et al. Pathways to achieve a set of ambitious global sustainability objectives by 2050: explorations using the IMAGE integrated assessment model. Technol. Forecast. Soc. Change 98, 303–323 (2015).
Google Scholar
-
van Vuuren, D. P. et al. Defining a sustainable development target space for 2030 and 2050. One Earth 5, 142–156 (2022).
Google Scholar
-
Soergel, B. et al. A sustainable development pathway for climate action within the UN 2030 Agenda. Nat. Clim. Change 11, 656–664 (2021).
Google Scholar
-
Campbell, B. et al. Agriculture production as a major driver of the Earth system exceeding planetary boundaries. Ecol. Soc. 22, 8 (2017).
Google Scholar
-
Springmann, M. et al. Options for keeping the food system within environmental limits. Nature 562, 519–525 (2018).
Google Scholar
-
Murray, C. J. L. The global burden of disease study at 30 years. Nat. Med. 28, 2019–2026 (2022).
Google Scholar
-
Conijn, J. G., Bindraban, P. S., Schröder, J. J. & Jongschaap, R. E. E. Can our global food system meet food demand within planetary boundaries? Agric. Ecosyst. Environ. 251, 244–256 (2018).
Google Scholar
-
Chrysafi, A. et al. Quantifying Earth system interactions for sustainable food production via expert elicitation. Nat. Sustain. 5, 830–842 (2022).
Google Scholar
-
Ruben, R., Cavatassi, R., Lipper, L., Smaling, E. & Winters, P. Towards food systems transformation — five paradigm shifts for healthy, inclusive and sustainable food systems. Food Secur. 13, 1423–1430 (2021).
Google Scholar
-
Fan, S. Economics in food systems transformation. Nat. Food 2, 218–219 (2021).
Google Scholar
-
Webb, P. et al. The urgency of food system transformation is now irrefutable. Nat. Food 1, 584–585 (2020).
Google Scholar
-
Sperling, F., Rumbaitis del Rio, C. & Laurien, F. Resilience and the Transformation of Food and Land Use Systems. Discussion paper prepared for the Food and Land Use Coalition (FOLU). https://iiasa.dev.local/ (2022).
-
Abrahão, G. et al. Transforming Human Systems to Safeguard the Global Commons: A Report by PIK and CGC. https://cgc.ifi.u-tokyo.ac.jp/wp-content/uploads/2024/03/GCS_report_2024.pdf (PIK/CGC, 2024).
-
Dixson-Declève, S. et al. Earth for All: A Survival Guide for Humanity (New Society, 2022).
-
Otto, I. M. et al. Social tipping dynamics for stabilizing Earth’s climate by 2050. Proc. Natl. Acad. Sci. USA 117, 2354–2365 (2020).
Google Scholar
-
Lenton, T. M. et al. (eds) The Global Tipping Points Report 2023 (Univ. Exeter, 2023).
-
Zipper, S. C. et al. Integrating the water planetary boundary with water management from local to global scales. Earths Future 8, e2019EF001377 (2020).
Google Scholar
-
Donges, J. F. et al. Taxonomies for structuring models for World–Earth systems analysis of the Anthropocene: subsystems, their interactions and social–ecological feedback loops. Earth Syst. Dyn. 12, 1115–1137 (2021).
Google Scholar
-
Anderies, J. et al. A modeling framework for World-Earth system resilience: exploring social inequality and Earth system tipping points. Environ. Res. Lett. https://doi.org/10.1088/1748-9326/ace91d (2023).
Google Scholar
-
Strnad, F. M., Barfuss, W., Donges, J. F. & Heitzig, J. Deep reinforcement learning in World-Earth system models to discover sustainable management strategies. Chaos Interdiscip. J. Nonlinear Sci. 29, 123122 (2019).
Google Scholar
-
Beckage, B., Moore, F. C. & Lacasse, K. Incorporating human behaviour into Earth system modelling. Nat. Hum. Behav. 6, 1493–1502 (2022).
Google Scholar
-
Qin, Y. et al. Carbon loss from forest degradation exceeds that from deforestation in the Brazilian Amazon. Nat. Clim. Change 11, 442–448 (2021).
Google Scholar
-
Rockström, J. et al. Identifying a safe and just corridor for people and the planet. Earths Future 9, e2020EF001866 (2021).
Google Scholar
Acknowledgements
This work was funded by the European Research Council through the grant ERC-2016-ADG-743080 (the Earth Resilience in the Anthropocene project). J.F.D. is grateful for financial support by the German Federal Ministry for Education and Research (BMBF) within the project ‘PIK_Change’ under grant 01LS2001A. L.W.E. further acknowledges financial support from Formas (2022-02089; 2019-01220), Horizon Europe (101081661) and the IKEA Foundation. The authors thank S. Cornell, who contributed advice and insights especially during the early stages of the manuscript, and L. Warszawski, who proofread the manuscript and provided advice. F. Pharand-Deschênes and J. Kaiser contributed to producing the figures.
Author information
Authors and Affiliations
Contributions
J.R. developed the concept for this Review. I.F. and M.A.M. researched the data for the illustrations. J.R., J.F.D., I.F., M.A.M., L.W.E. and K.R. substantially contributed to the discussion of the content. J.R., J.F.D., M.A.M., L.W.E. and K.R. participated in writing and reviewing/editing the manuscript before submission.
Corresponding authors
Ethics declarations
Competing interests
The authors declare no competing interests.
Peer review
Peer review information
Nature Reviews Earth & Environment thanks Andrew Fanning, Xuemei Bai and the other, anonymous, reviewer(s) for their contribution to the peer review of this work.
Additional information
Publisher’s note Springer Nature remains neutral with regard to jurisdictional claims in published maps and institutional affiliations.
Supplementary information
Supplementary information
Rights and permissions
Springer Nature or its licensor (e.g. a society or other partner) holds exclusive rights to this article under a publishing agreement with the author(s) or other rightsholder(s); author self-archiving of the accepted manuscript version of this article is solely governed by the terms of such publishing agreement and applicable law.
Reprints and permissions
About this article
Cite this article
Rockström, J., Donges, J.F., Fetzer, I. et al. Planetary Boundaries guide humanity’s future on Earth.
Nat Rev Earth Environ 5, 773–788 (2024). https://doi.org/10.1038/s43017-024-00597-z
-
Accepted: 06 September 2024
-
Published: 08 November 2024
-
Issue Date: November 2024
-
DOI: https://doi.org/10.1038/s43017-024-00597-z